Dark matter first originated in a ‘Dark Big Bang’, study finds
Dark matter, though invisible, weighs heavily on how we understand the universe. Its gravity sculpts galaxies, holds clusters together, and shapes cosmic evolution.
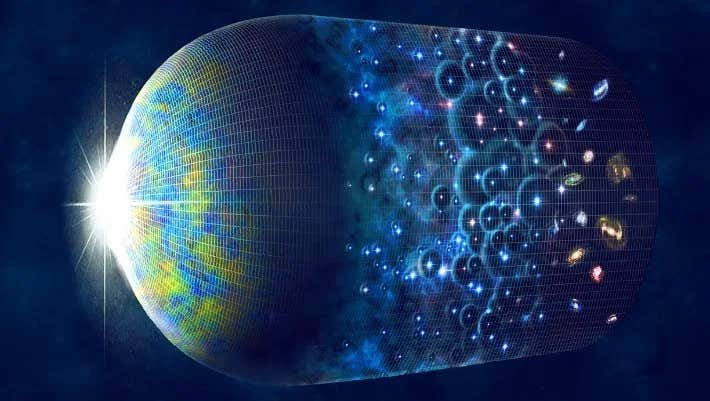
Dark matter first entered the scientific spotlight in the 1930s, when astronomers noticed that galaxy clusters were moving in ways that couldn’t be explained by the visible matter alone. (CREDIT: CfA/M. Weiss)
Dark matter, though invisible, weighs heavily on how we understand the universe. Its gravity sculpts galaxies, holds clusters together, and shapes cosmic evolution—yet we still don’t know what it is. Nearly 100 years after its effects were first noticed, it remains one of the most puzzling forces in physics.
This mystery traces back to the 1930s. Astronomers then saw that galaxies in clusters were moving in ways that didn’t make sense. The visible matter wasn’t enough to explain the speeds. Something unseen had to be there, exerting a hidden pull: what we now call dark matter.
Support for dark matter deepened decades later with the study of the cosmic microwave background—the faint radiation left over from the Big Bang. These patterns in the CMB revealed how invisible mass shaped the early universe, making dark matter crucial in cosmological models.
By 2018, the Planck Collaboration estimated that dark matter accounts for 27% of the universe’s energy. That dwarfs the 5% made of familiar atoms and molecules. Despite this dominance, dark matter continues to resist detection.
To uncover its nature, scientists have turned to bold theories. One is supersymmetry, or SUSY, a proposed extension of the Standard Model. SUSY predicts that each known particle has a hidden partner. Among these, some could make up dark matter.
That led researchers to the idea of weakly interacting massive particles, or WIMPs. These particles, if real, would barely touch ordinary matter, making them hard to find. Hopes were pinned on finding them in powerful colliders like the Large Hadron Collider (LHC) or in deep underground detectors.
So far, WIMPs have stayed silent. DAMA, an experiment that claimed to see a yearly signal possibly tied to dark matter, sparked excitement—and controversy. Other projects like COSINE-100 tried to repeat the result but couldn’t confirm it. Meanwhile, the LHC has yet to spot any SUSY particles, forcing physicists to rethink their models.
In 2023, a fresh theory arrived: the Dark Big Bang. Katherine Freese and Martin Winkler at the University of Texas at Austin proposed it. Unlike the classic Big Bang that explains visible matter, the DBB suggests dark matter came from a separate event entirely—one that shaped a shadow universe with its own origin story.
Related Stories
This second Big Bang, occurring sometime after the first, would have generated dark matter through the decay of a quantum field trapped in a false vacuum state.
In this model, the early universe consisted of two sectors: the visible sector, filled with the familiar particles and forces, and a dark sector, which remained cold and decoupled. Eventually, the dark sector underwent its own phase transition, analogous to the visible sector’s hot Big Bang.
This transition produced a thermal bath of dark particles, governed by a unique set of physical laws. The DBB model is particularly versatile, as it can accommodate a wide range of dark matter particle masses, from as light as a few keV to as heavy as 101210^{12}1012 GeV.
What sets the DBB model apart is its potential to leave observable traces. The phase transition in the dark sector could generate gravitational waves (GWs), ripples in the fabric of spacetime. These GWs would be distinct from those produced by black hole mergers or neutron star collisions and could be detected by next-generation observatories.
In particular, low-frequency GWs detectable by pulsar timing arrays (PTAs) such as the International Pulsar Timing Array (IPTA) and the Square Kilometer Array (SKA) could provide crucial evidence for the DBB.
Recent work by Cosmin Ilie, an Assistant Professor of Physics and Astronomy at Colgate University, and Richard Casey, a senior physics student, has further refined the DBB theory. Their study explores new parameter spaces for the dark sector's tunneling field, identifying scenarios that align with existing cosmological observations.
These scenarios predict not only the correct abundance of dark matter but also GW signals that could soon be within reach of PTA experiments.
“Detecting gravitational waves generated by the Dark Big Bang could provide crucial evidence for this new theory of dark matter,” says Ilie. Such detection would be groundbreaking, offering the first direct evidence of dark matter's distinct origin.
The 2023 detection of background GWs by the NANOGrav collaboration, a part of IPTA, adds an intriguing dimension to this research. While the exact source of these waves remains uncertain, they could potentially align with the DBB model’s predictions.
Beyond its implications for dark matter, the DBB theory offers a fresh perspective on the early universe. Traditionally, cosmology has operated under the assumption that all matter, dark or otherwise, emerged from the same event.
The idea of a dual-origin universe challenges this notion, suggesting a more complex interplay of forces and fields in the universe's infancy. If confirmed, the DBB model could reshape our understanding of cosmic evolution, from the formation of the first galaxies to the large-scale structure of the universe.
The search for dark matter is a central pillar of modern physics, driving advancements in technology and theory. Direct detection experiments, such as those conducted deep underground, continue to push the boundaries of sensitivity, aiming to capture fleeting interactions between dark matter particles and ordinary matter.
Meanwhile, astrophysical observations, from the CMB to galactic rotation curves, provide indirect but compelling evidence for dark matter’s gravitational influence. The DBB model, with its unique predictions and testable consequences, adds a powerful new tool to this arsenal.
As observational capabilities advance, the prospect of detecting GWs from a DBB becomes increasingly plausible. Projects like SKA, expected to come online in the next decade, promise unprecedented sensitivity to low-frequency GWs. These efforts could finally lift the veil on dark matter's mysterious origins, answering questions that have puzzled scientists for generations.
In the broader context, understanding dark matter is not just a scientific pursuit but a quest to comprehend the fundamental nature of the universe. Whether through traditional particle physics or novel cosmological theories like the DBB, each discovery brings us closer to unveiling the full tapestry of existence.
Note: Materials provided above by The Brighter Side of News. Content may be edited for style and length.
Like these kind of feel good stories? Get The Brighter Side of News' newsletter.
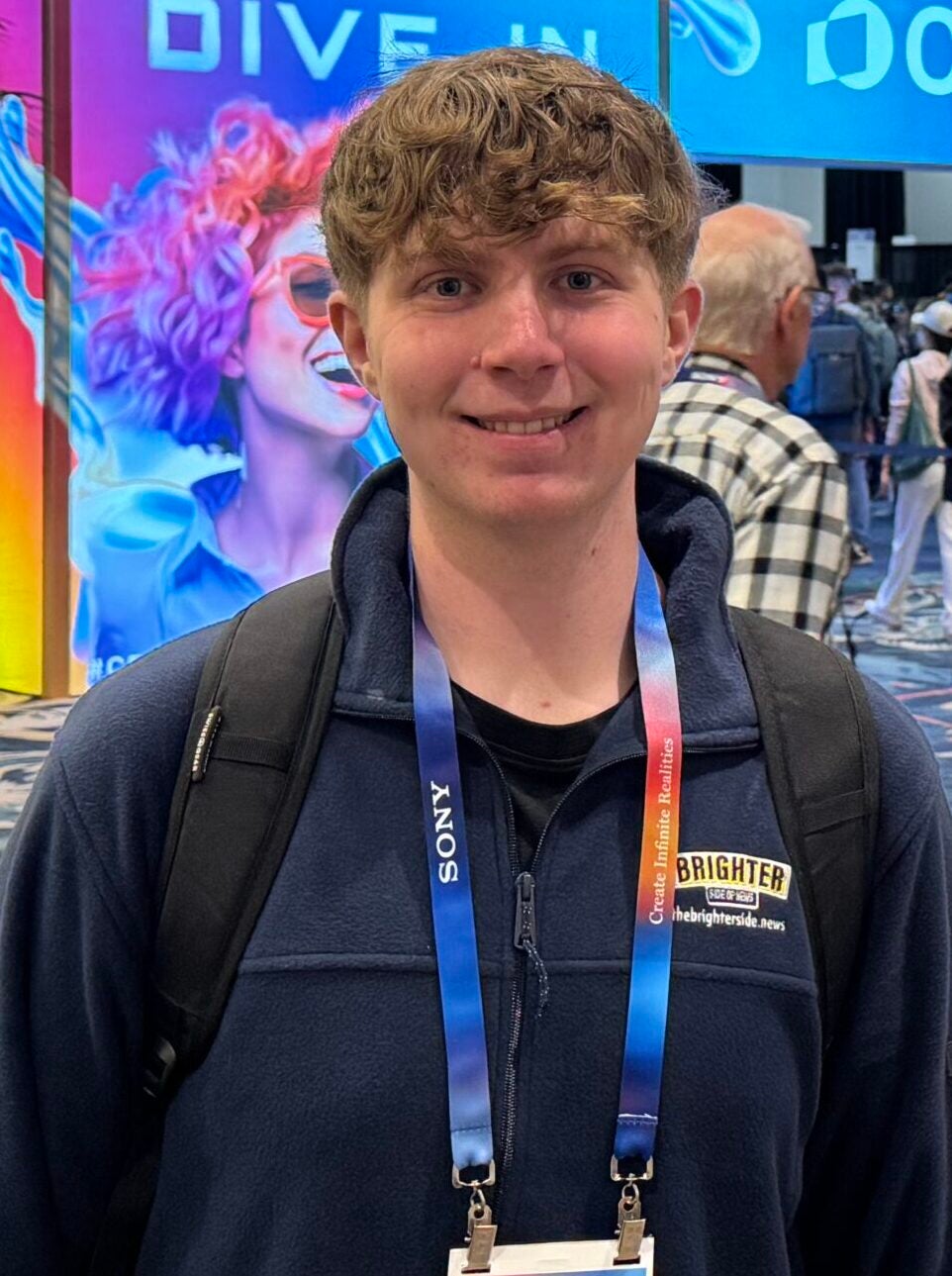
Joshua Shavit
Science & Technology Writer | AI and Robotics Reporter
Joshua Shavit is a Los Angeles-based science and technology writer with a passion for exploring the breakthroughs shaping the future. As a contributor to The Brighter Side of News, he focuses on positive and transformative advancements in AI, technology, physics, engineering, robotics and space science. Joshua is currently working towards a Bachelor of Science in Business Administration at the University of California, Berkeley. He combines his academic background with a talent for storytelling, making complex scientific discoveries engaging and accessible. His work highlights the innovators behind the ideas, bringing readers closer to the people driving progress.