Groundbreaking study solves the mystery of how our brain learns
Neuroscientists uncover how brain cells use multiple synaptic plasticity rules simultaneously, reshaping our understanding of learning.
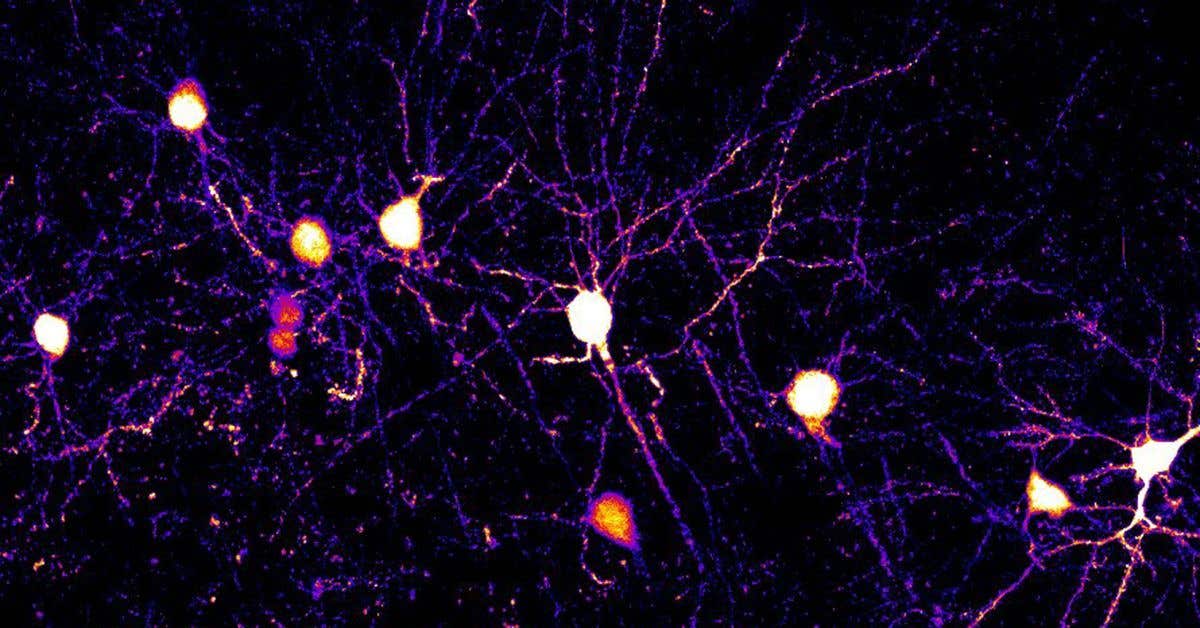
Neurons and their branch extensions known as dendrites are featured within a mouse’s cerebral cortex. (CREDIT: UCSD)
Learning is something everyone does daily—mastering new skills at work, remembering song lyrics, or following directions to new places. But behind these everyday tasks lies a complex biological process known as synaptic plasticity, a phenomenon essential for your brain's ability to adapt and store new information.
Recently, neuroscientists have discovered surprising new details about how your brain decides which synapses—the tiny connections between nerve cells—get stronger or weaker as you learn. These insights challenge earlier ideas, revealing that your brain applies multiple rules simultaneously to reshape its connections during learning.
The Mystery of Synaptic Plasticity
Your brain consists of billions of nerve cells, or neurons, interconnected by synapses. When you learn, specific synapses grow stronger, while others become weaker, adapting your brain's circuitry to new tasks or information.
Until recently, scientists thought that neurons used uniform rules for synaptic changes. Yet, precisely how each neuron selects the synapses for strengthening or weakening remained a scientific puzzle—known as the "credit assignment problem." Understanding this puzzle is crucial because it determines how your brain records and recalls learned information.
William “Jake” Wright, Nathan Hedrick, and Takaki Komiyama, neurobiologists at the University of California San Diego, tackled this puzzle head-on. Using advanced brain imaging methods, their groundbreaking research revealed that neurons don't follow a single rule when learning. Instead, neurons use multiple different rules simultaneously. Their findings, published in the journal Science, have profound implications for neuroscience, medicine, and even artificial intelligence.
A Closer Look into the Brain's Learning Rules
To uncover these new insights, the researchers observed how synapses behaved during motor learning tasks in mice. Using two-photon imaging—a powerful method allowing scientists to visualize brain activity in real-time—they tracked individual synapses within layer 2/3 pyramidal neurons in the motor cortex.
Related Stories
These neurons have complex structures, branching into apical dendrites (extending upward) and basal dendrites (extending sideways or downward). Surprisingly, each dendrite type followed different rules for synaptic strengthening during learning.
Specifically, the team found that synapses on apical dendrites became stronger when activated alongside neighboring synapses. These synapses respond collectively to local activity patterns, strengthening connections based on what's happening nearby.
On the other hand, basal dendrite synapses strengthened when their activity coincided with action potentials—brief electrical signals traveling down neurons. In short, the apical synapses responded to neighborhood activity, while basal synapses depended on precise timing with neuron signals.
“We usually think of synaptic plasticity as uniform throughout the brain,” explained Wright, lead author of the study. “But now we see clearly that neurons use distinct rules for synaptic plasticity in different parts of their dendritic branches.”
This discovery came unexpectedly, prompting scientists to reconsider previous assumptions. Until now, research mostly indicated that synapses across neurons followed a single, uniform rule—commonly called Hebbian plasticity—where connections strengthen if both sides of a synapse activate simultaneously.
While Hebbian plasticity explains many learning processes, scientists have long suspected additional, more nuanced mechanisms exist. This study confirmed those suspicions, revealing how neurons simultaneously apply multiple plasticity rules in a compartment-specific manner.
Implications Beyond Neuroscience
The implications of these findings reach far beyond basic brain science. The discovery fundamentally changes how researchers understand the "credit assignment problem." Each synapse operates like an individual worker in a vast factory, contributing without seeing the bigger picture.
Yet, neurons manage to coordinate thousands of these workers simultaneously, using different rules depending on location within the neuron. This complex system could explain the brain's remarkable flexibility in learning various tasks simultaneously.
According to senior author Komiyama, these findings significantly advance the understanding of brain function. "This discovery fundamentally changes the way we understand how the brain solves the credit assignment problem," said Komiyama. "Individual neurons perform distinct computations in parallel, using different rules within different cellular compartments."
Beyond biology, this research offers crucial insights for artificial intelligence. Current AI systems use neural networks inspired by brain structure but typically apply uniform rules across their entire network. Understanding how your brain employs multiple rules simultaneously could inspire new, more powerful AI designs.
Scientists envision creating neural networks with greater flexibility and learning efficiency by mimicking the brain's strategy of compartment-specific plasticity.
Hope for Health and Disease Treatment
The researchers emphasize the potential health impacts of understanding synaptic plasticity better. Many neurological and psychological disorders, such as Alzheimer's disease, addiction, autism, and PTSD, involve dysfunctions in synaptic communication. Knowing how healthy brains selectively strengthen or weaken synapses might lead scientists toward targeted treatments for these conditions.
“Our research provides a clearer understanding of how synapses are modified during learning,” Wright explained. “This could have important health implications since many diseases involve synaptic dysfunction.”
Scientists hope this foundational knowledge will aid in identifying precisely what's going wrong at the synaptic level during disorders like autism or Alzheimer’s. It might lead to therapies that correct specific synaptic imbalances, offering improved treatments for people living with these challenging conditions.
What's Next for Neuroscientists?
Following this discovery, the research team plans to explore further how neurons can simultaneously apply multiple rules. Future studies will delve deeper into the biochemical and molecular processes behind compartment-specific plasticity. Researchers also aim to identify why using multiple rules provides an advantage in learning new information or behaviors.
Ultimately, their goal is to unlock more profound knowledge about brain functionality, supporting advancements in medicine, education, and technology. By understanding how neurons manage complex computations effortlessly, scientists can open doors to exciting new possibilities in treating brain diseases, developing sophisticated artificial intelligence, and enhancing overall understanding of human behavior.
“Our findings have provided a foundation for exploring how the brain normally works,” Wright concluded. “Now, we want to understand exactly how neurons benefit from using different rules simultaneously.”
Note: The article above provided above by The Brighter Side of News.
Like these kind of feel good stories? Get The Brighter Side of News' newsletter.
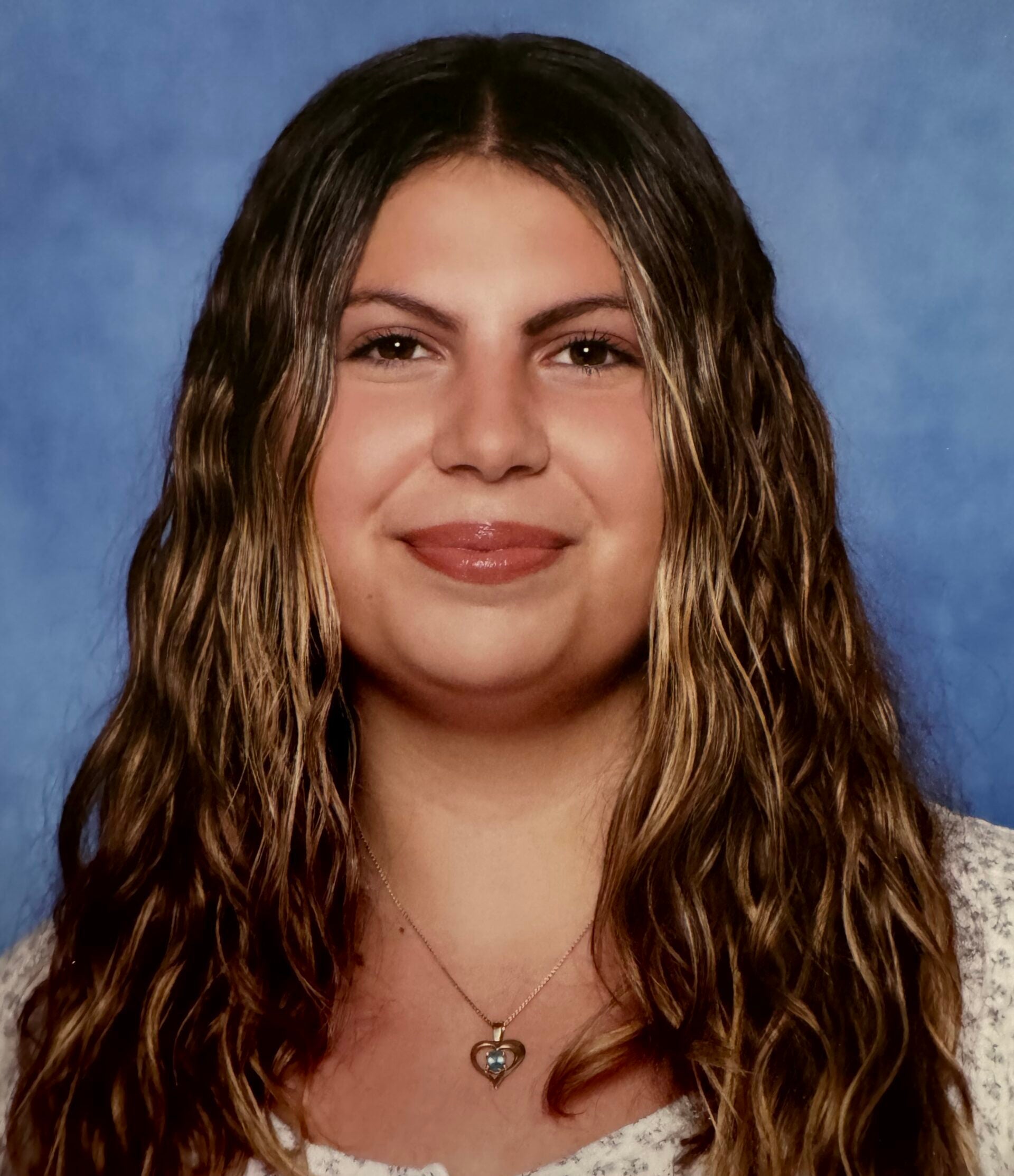
Rebecca Shavit
Science & Technology Journalist | Innovation Storyteller
Based in Los Angeles, Rebecca Shavit is a dedicated science and technology journalist who writes for The Brighter Side of News, an online publication committed to highlighting positive and transformative stories from around the world. With a passion for uncovering groundbreaking discoveries and innovations, she brings to light the scientific advancements shaping a better future. Her reporting spans a wide range of topics, from cutting-edge medical breakthroughs and artificial intelligence to green technology and space exploration. With a keen ability to translate complex concepts into engaging and accessible stories, she makes science and innovation relatable to a broad audience.