Groundbreaking technology locks away atmospheric CO2 into solid carbon nanofibers for long-term use
This inventive approach not only locks away carbon in a useful solid form but also produces hydrogen gas, a zero-emission alternative fuel
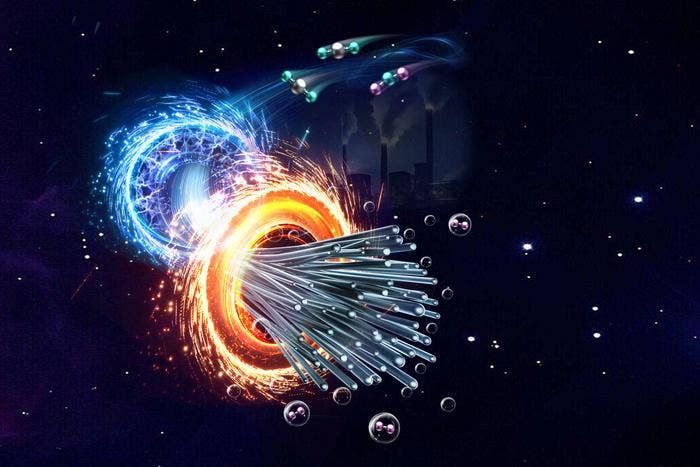
Researchers have devised a revolutionary method to combat climate change by converting carbon dioxide (CO2), a potent greenhouse gas, into valuable carbon nanofibers (CNF). (CREDIT: Creative Commons)
In a groundbreaking development, scientists from the U.S. Department of Energy's (DOE) Brookhaven National Laboratory and Columbia University have devised a revolutionary method to combat climate change by converting carbon dioxide (CO2), a potent greenhouse gas, into valuable carbon nanofibers (CNF).
This remarkable strategy harnesses tandem electrochemical and thermochemical reactions, all performed at relatively low temperatures and ambient pressure. As reported in the prestigious journal Nature Catalysis, this inventive approach not only locks away carbon in a useful solid form but also produces hydrogen gas (H2), a zero-emission alternative fuel.
Carbon nanofibers, characterized by their extraordinary properties such as strength, thermal conductivity, and electrical conductivity, have long been recognized as a material with vast potential.
However, extracting carbon from carbon dioxide and manipulating it to form these fine-scale structures has proven to be a formidable challenge. Previous attempts required exceedingly high temperatures exceeding 1,000 degrees Celsius, rendering large-scale carbon dioxide mitigation impractical.
Related Stories
Jingguang Chen, a professor of chemical engineering at Columbia University and a researcher at Brookhaven Lab, led the study and emphasized the significance of their achievement: "It's very unrealistic for large-scale CO2 mitigation to rely on high-temperature processes. In contrast, we found a process that can occur at about 400 degrees Celsius, which is a much more practical, industrially achievable temperature."
The key to their success lay in breaking down the conversion process into stages and employing two distinct catalysts, each optimized for its respective role. This innovative approach, known as the tandem two-step process, holds the potential to revolutionize carbon dioxide utilization.
The scientists' journey towards this groundbreaking discovery began with the realization that carbon monoxide (CO) served as a superior starting material for producing carbon nanofibers compared to CO2. They then embarked on determining the most efficient means of generating CO from CO2.
The electrocatalytic-thermocatalytic tandem strategy for CNF production circumvents thermodynamic constraints by combining the co-electrolysis of CO2 and water into syngas (CO and H2) with a subsequent thermochemical process under mild conditions (370-450 °C, ambient pressure). (CREDIT: Zhenhua Xie/Brookhaven National Laboratory and Columbia University)
Their investigation led them to employ a commercially available electrocatalyst comprised of palladium supported on carbon. This electrocatalyst facilitated the splitting of both CO2 and water (H2O) into CO and H2 in the presence of flowing electrons and protons. Subsequently, they introduced a heat-activated thermocatalyst composed of an iron-cobalt alloy, capable of operating at the moderate temperature of around 400 degrees Celsius. Adding a small amount of metallic cobalt further enhanced the formation of carbon nanofibers.
Chen elaborated on the significance of combining electrocatalysis and thermocatalysis, stating, "By coupling electrocatalysis and thermocatalysis, we are using this tandem process to achieve things that cannot be achieved by either process alone."
To gain insight into the intricate workings of their catalysts, the researchers conducted a multitude of experiments, including computational modeling studies, physical and chemical characterizations, and microscopic imaging. These investigations were conducted at Brookhaven Lab's National Synchrotron Light Source II (NSLS-II) and the Electron Microscopy facility at the Lab's Center for Functional Nanomaterials (CFN).
Computational modeling, specifically "density functional theory" (DFT) calculations, allowed the scientists to scrutinize the atomic structures and interactions within the catalysts during chemical reactions. These calculations revealed the stable phases of the catalysts under reaction conditions, the active sites involved, and the barriers or transition states between reaction steps, shedding light on the catalyst's function.
At NSLS-II, x-ray diffraction and x-ray absorption experiments tracked the physical and chemical transformations of the catalysts during the reactions. These experiments provided crucial insights into how metallic palladium in the catalyst changed into palladium hydride when subjected to an electric current, a transformation pivotal for producing both CO and H2.
In the second stage of the reaction, researchers delved into the structure of the iron-cobalt system under reaction conditions and sought to optimize the iron-cobalt catalyst. X-ray experiments confirmed that an alloy of iron and cobalt, along with additional metallic cobalt, was essential for converting CO into carbon nanofibers.
The synergy between the two catalysts became apparent. According to Liu, "The cobalt-iron sites in the alloy help to break the C-O bonds of carbon monoxide, making atomic carbon available to serve as the source for building carbon nanofibers. Then the extra cobalt is there to facilitate the formation of the C-C bonds that link up the carbon atoms."
Recycling Catalysts and Carbon-Negative Potential
One of the most striking advantages of this process is the ease with which the catalysts can be recycled. As the carbon nanofibers grow, the catalyst gets pushed up and away from the surface, facilitating its extraction and reuse. Chen explained, "We use acid to leach the metal out without destroying the carbon nanofiber so we can concentrate the metals and recycle them to be used as a catalyst again."
High-resolution transmission electron microscopy (TEM) shows the tip of the resulting carbon nanofiber (left) on the iron-cobalt/cerium oxide (FeCo/CeO2) thermocatalyst. (CREDIT: Center for Functional Nanomaterials/Brookhaven National Laboratory)
This recycling capability, coupled with the commercial availability of the catalysts and the relatively mild reaction conditions for the second stage, contributes to a favorable assessment of the energy and cost implications associated with the process.
Chen highlighted the importance of this research for practical applications, stating, "Our technical results and these other analyses show that this tandem strategy opens a door for decarbonizing CO2 into valuable solid carbon products while producing renewable H2."
Electrochemical–thermochemical tandem process for producing CNFs and renewable H2. (CREDIT: Zhenhua Xie/Brookhaven National Laboratory and Columbia University)
Furthermore, when powered by renewable energy sources, the entire process holds the promise of being carbon-negative, marking a significant stride toward effective CO2 mitigation. This groundbreaking research not only offers a solution to combat climate change but also opens new possibilities for sustainable carbon utilization and renewable energy production.
With the ability to lock carbon away in solid form, recycle catalysts, and produce renewable hydrogen, this breakthrough offers a promising solution to combat climate change while ushering in new opportunities for renewable energy and carbon-negative technologies.
For more science and technology news stories check out our New Innovations section at The Brighter Side of News.
Note: Materials provided above by The Brighter Side of News. Content may be edited for style and length.
Like these kind of feel good stories? Get the Brighter Side of News' newsletter.
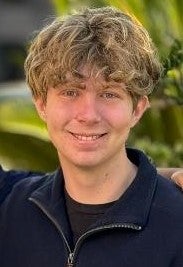