MIT scientists capture first images of quantum interactions
MIT researchers created a quantum microscope, capturing the first images of atoms interacting freely.
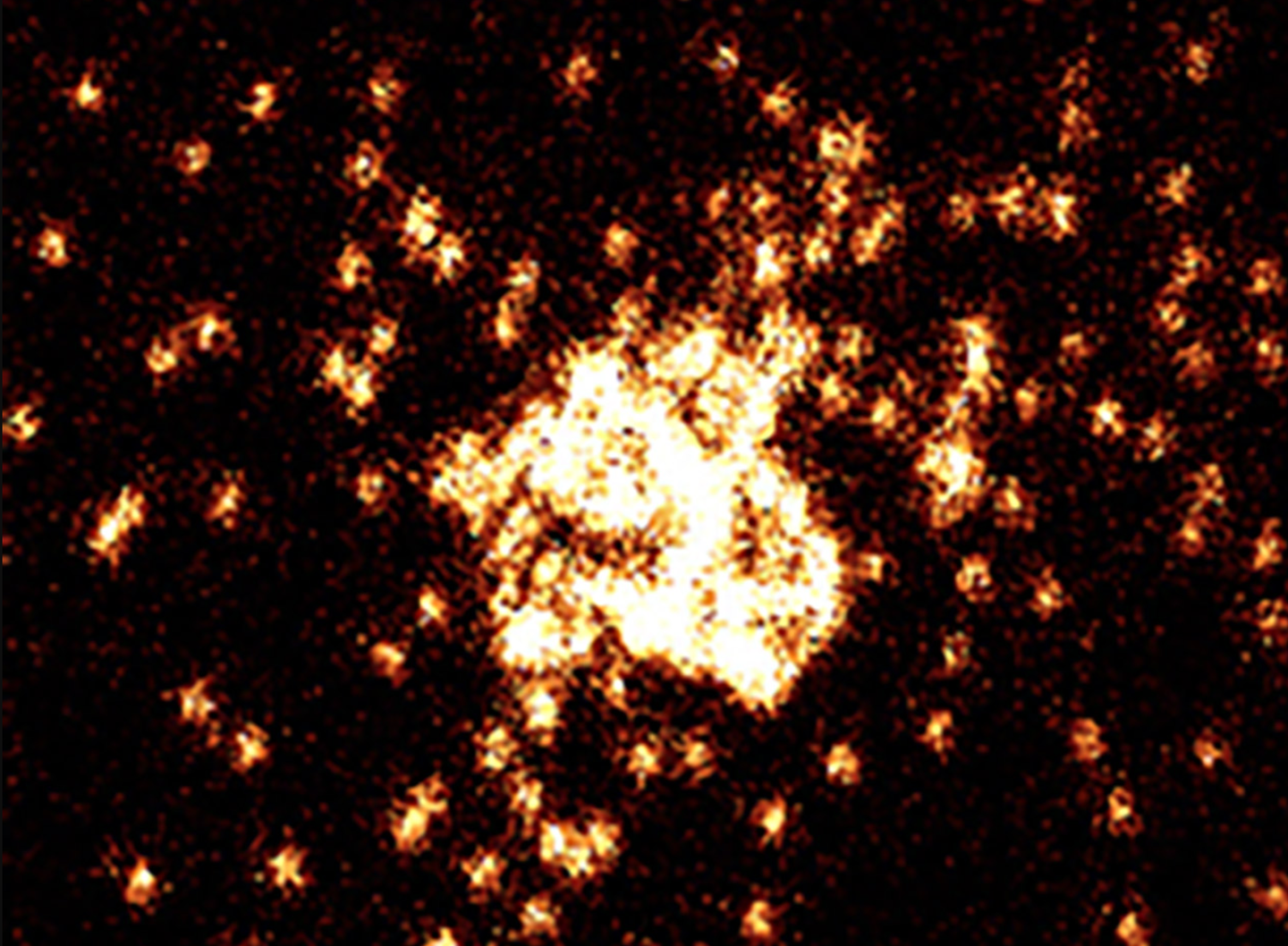
MIT physicists reveal first direct images of atoms interacting freely, unlocking unseen quantum phenomena. (CREDIT: Physical Review Letters)
MIT physicists have taken the first-ever direct images of individual atoms interacting freely in space. Their findings, published in the journal Physical Review Letters, reveal hidden quantum behaviors that scientists previously could only predict mathematically. These insights could change how we understand quantum physics and lead to new ways of exploring matter at its most basic level.
Seeing Quantum Particles Clearly
Atoms, tiny building blocks of matter, are tough to observe directly. They are about one-tenth of a nanometer wide—one-millionth the width of human hair. Unlike everyday objects, atoms follow quantum mechanics, behaving more like waves than solid particles. Before this study, scientists could only broadly view clouds of atoms using indirect methods. It was like seeing clouds in the sky without seeing individual water droplets.
MIT physicist Martin Zwierlein and his team developed a new imaging method to solve this issue. First, they trapped a cloud of atoms loosely in a space created by laser beams. This setup allowed atoms to freely interact, mimicking their natural environment. Then the researchers quickly switched on a "light lattice" to freeze atoms instantly in place. Finally, a second laser illuminated the atoms, and their fluorescence—the tiny flashes of light they emitted—revealed their exact positions.
“The hardest part was to gather the light from the atoms without boiling them out of the optical lattice,” explains Zwierlein. "We’ve learned some tricks through the years. It’s the first time we can freeze atoms when they’re strongly interacting and see them individually."
Revealing Bosons and Fermions
The MIT team imaged two different types of atoms, known scientifically as bosons and fermions. These types differ in their quantum spin, a fundamental property determined by how their internal particles—protons, neutrons, and electrons—are counted. Bosons tend to group together, while fermions naturally repel each other.
First, the researchers observed bosons made from sodium atoms. When cooled close to absolute zero, these atoms form a special state called a Bose-Einstein condensate. In this state, bosons behave collectively as one big wave, predicted nearly a century ago by physicist Louis de Broglie. Directly imaging this phenomenon showed bosons clearly bunched together.
Related Stories
"We understand much more from their wave-like nature," Zwierlein says. "It’s usually very tough to observe these quantum effects. Our new microscope lets us visualize this wave directly."
Next, they studied fermions using lithium atoms. Fermions typically avoid each other, creating what physicists call a "Fermi hole"—a gap that keeps identical particles apart.
However, the researchers saw something extraordinary. Different types of fermions actually paired up closely, forming strong quantum bonds. These pairs are fundamental in superconductivity, where materials conduct electricity without resistance.
"This kind of pairing is the basis of a mathematical construction people developed to explain experiments," says co-author Richard Fletcher. "But when you see pictures like these, it brings mathematical ideas to life. It's a powerful reminder that physics is about physical reality."
Quantum Simulation in Action
Quantum physics studies how particles behave together. Sometimes, particles interact simply because of their quantum nature; other times, interactions occur due to direct contact. These interactions often create complex situations difficult for scientists to predict.
This difficulty has spurred the development of quantum simulations. In these setups, ultracold gases mimic more complex quantum systems. Quantum simulations have enabled researchers to explore phenomena like high-temperature superconductors and quantum magnets. Previously, scientists used "lattice" setups, trapping atoms in grid-like structures to study particle interactions.
The MIT team's method significantly advances these simulations. Unlike past setups limited to lattices, this technique observes atoms moving freely in space. It reveals details previously hidden by coarser imaging methods. Scientists can now measure precise properties like particle correlations, density, pressure, and temperature directly within the cloud of atoms.
"Our technique can open doors to new experiments exploring strongly correlated quantum gases," Zwierlein emphasizes. "It allows us to study mixtures of bosons and fermions, exotic quantum phases, and particles like dipolar atoms and quantum polarons."
Capturing Quantum Phenomena
To achieve their breakthrough, researchers used a "pinning lattice" created by a specialized laser setup. This method briefly locked the atoms in a pattern, allowing precise imaging. Each atom appeared clearly distinct, separated by less than a micrometer—thousands of times smaller than a human hair.
This precision revealed "two-particle correlations," a critical feature in quantum physics. For instance, bosons showed enhanced correlations, clearly visible as bunched groups. Fermions showed suppressed correlations—the famous "Fermi hole." By adjusting interactions, researchers could directly see fermion pairs form, observing phenomena known as the BEC-BCS crossover.
Their work also showcased the ability to measure fundamental properties of these pairs. Scientists captured the "pairing gap," showing how tightly fermions paired. They measured "pair size" and a feature called "short-range contact," critical for understanding strong quantum interactions.
Looking Ahead to Quantum Discoveries
Zwierlein's team plans to use their groundbreaking microscope to explore even stranger quantum behaviors, such as quantum Hall physics. These situations, which occur under strong magnetic fields, produce states so complex that scientists still struggle to explain them clearly.
"That’s where theory gets really tricky," Zwierlein notes. "People draw rough pictures because full theories can't easily solve these problems. Our microscope lets us verify if these strange quantum states are actually real."
Through their innovative imaging technique, MIT physicists have bridged the gap between quantum theory and visible reality. Their groundbreaking work provides a clearer window into the quantum world, helping scientists uncover mysteries that could reshape how we understand the very fabric of matter.
Note: The article above provided above by The Brighter Side of News.
Like these kind of feel good stories? Get The Brighter Side of News' newsletter.
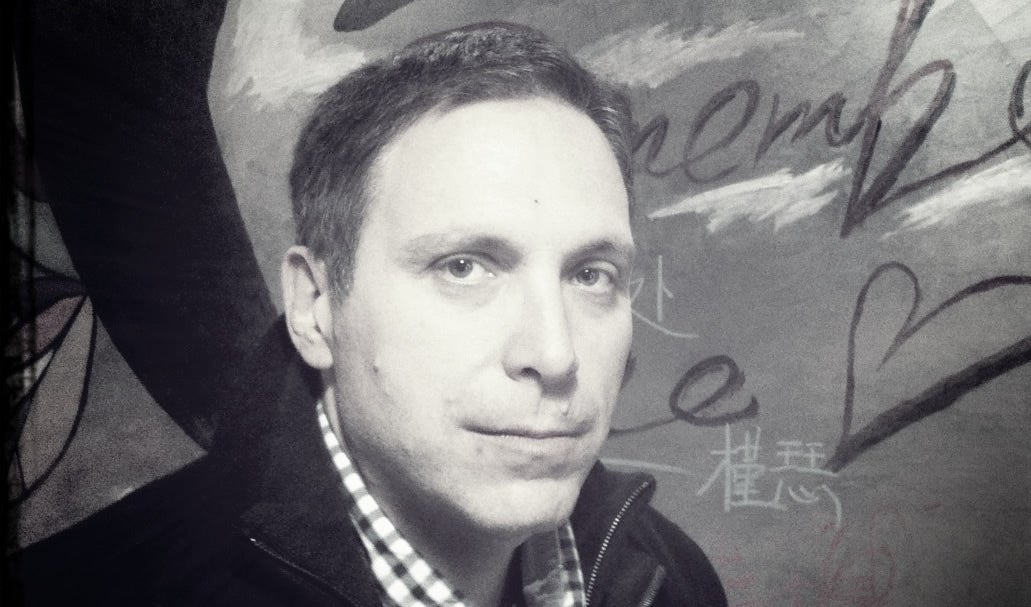
Joseph Shavit
Head Science News Writer | Communicating Innovation & Discovery
Based in Los Angeles, Joseph Shavit is an accomplished science journalist, head science news writer and co-founder at The Brighter Side of News, where he translates cutting-edge discoveries into compelling stories for a broad audience. With a strong background spanning science, business, product management, media leadership, and entrepreneurship, Joseph brings a unique perspective to science communication. His expertise allows him to uncover the intersection of technological advancements and market potential, shedding light on how groundbreaking research evolves into transformative products and industries.