New research key to developing sturdier construction material and more climate efficient trees
A genetic engineering method makes it possible to observe how woody cell walls are built in plants.
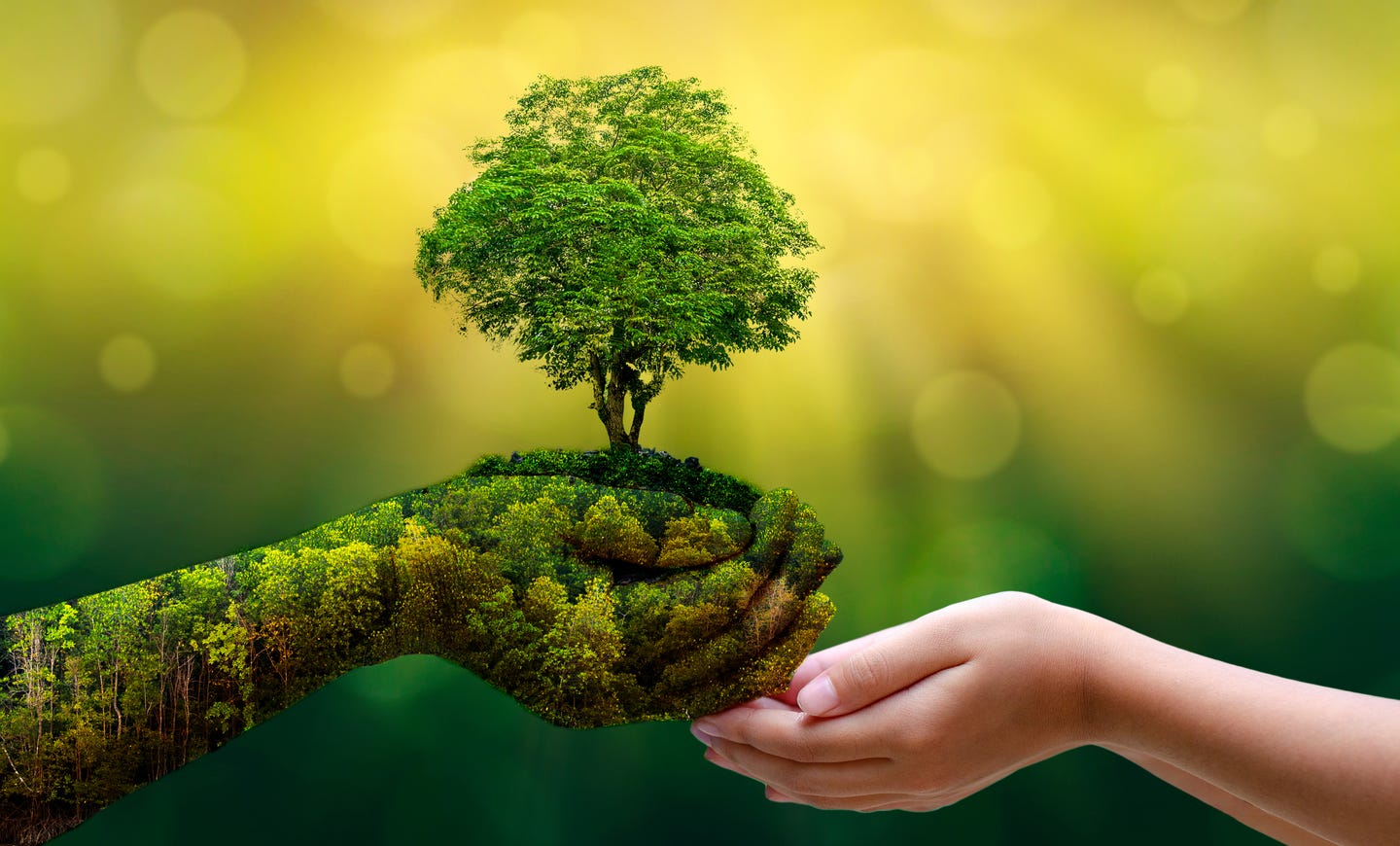
[Jan. 28, 2021: University of Copenhagen]
A genetic engineering method makes it possible to observe how woody cell walls are built in plants. The new research in wood formation, conducted by the University of Copenhagen and others, opens up the possibility of developing sturdier construction materials and perhaps more climate efficient trees.
The ability of certain tree species to grow taller than 100 meters is due to complex biological engineering. Besides needing the right amounts of water and light to do so, this incredible ability is also a result of cell walls built sturdily enough to keep a tree both upright and able to withstand the tremendous pressure created as water is sucked up from its roots and into its leaves.
This ability is made possible by what are known as the secondary cell walls of plants. Secondary cell walls, also known as xylem or wood, are built according to a few distinct and refined patterns that allow wall strength to be maintained while still allowing connecting cells to transport water from one to the other.
How these wall patterns are built has been a bit of a mystery. Now, the mystery is starting to resolve. For the first time, it is possible to observe the process of woody cell wall pattern formation within a plant--and in real-time no less. A team of international researchers, including Professor Staffan Persson of the University of Copenhagen, has found a way to monitor this biological process live, under the microscope. The scientific article for the study is published in the renowned journal, Nature Communications.
This opens up the possibility of manipulating the building process and perhaps even making plant xylem stronger--a point that shall be returned to.
A genetic trick makes it possible to observe wood formation in real-time
Because wood forms in tissue that is buried deep inside the plant, and microscopes work best on the surfaces of objects, the wood-forming process is difficult to observe. To witness it in action, researchers needed to apply a genetic trick. By modifying the plants with a genetic switch, they were able to turn on wood formation in all cells of the plant--including those on the surface. This allowed them to observe the wood formation under a microscope in detail and in real time.
Cell walls consist mainly of cellulose, which is produced by enzymes located on the surface of all plant cells. Generally speaking, the process involves the orderly arrangement of protein tubes - known as microtubules - at the surface of the cells. The microtubules serve as tracks along which the wall producing enzymes deposit construction material to the wall.
"One can imagine the construction process as a train network, where the train would represent the cellulose-producing enzymes, as they move forward while producing cellulose fibers. The microtubules, or rails, steer the direction of the proteins, just like train rails. Interestingly, during the formation of woody cell walls these "rails" need to change their organization completely to make patterned walls - a process that we now can follow directly under our microscopes" explains Staffan Persson, of UCPH's Department of Plant and Environmental Sciences.
Taller trees and sturdier building materials
"We now have a better understanding of the mechanisms that cause the microtubules to rearrange and form the patterns. Furthermore, we can simulate the wall pattern formation on a computer. The next step is to identify ways that allows us to make changes to the system. For example, by changing patterns," suggests Persson.
First author, Dr Rene Schneider chimes in:
"Changing the patterns can alter the ways in which a plant grows or distributes water within it, which can then go on to influence a plant's height or biomass. For example, if you could create trees that grow differently by accumulating more biomass, it could potentially help slow the increase in carbon dioxide in the atmosphere."
In the longer term, Persson suggests that one of the most obvious applications is to manipulate biological processes to develop stronger or different woody construction materials.
"If we can change both the chemical composition of cell walls, which we and other researchers are already working on, and the patterns of cell walls, we can probably alter wood strength and porosity. Sturdier construction materials made of wood don't just benefit the construction industry, but the environment and climate as well. They have a smaller carbon footprint, a longer service life and can be used for manifold purposes. In some cases, they may even be able to replace energy-intensive materials like concrete," says Persson, whose background as a construction engineer compliments his plant biology background.
He also points to potential applications in the development of cellulose-based nanomaterials. These are gaining ground in medicine, where cellulose-based nanomaterials can be used to efficiently transport pharmaceuticals throughout the body.
However, these applications would first require further knowledge in how we can manipulate secondary walls and implementation of the knowledge in trees or other useful crop plants as much of our research is conducted in model plant organisms, such as thale cress, underscores both Staffan Persson and Rene Schneider.
FACTS:
The secondary cell walls of plants are located between the primary cell wall and the cell surface. They are typically formed when the thinner primary cell wall stops growing. In addition to cellulose, one of the main components of cell wall is lignin, which fills in the cavities in cellulose and thereby strengthens the wall even more.
Secondary cell walls have two major patterns in two distinct stages of cell life. The first is a spiral pattern which twists around the cell, making the walls stronger and allowing for the cells to continue to grow. The second pattern is perforated by numerous small holes. The latter pattern doesn't allow for cell growth. However, as cells connect with each via the holes, they are able to transport water from one cell to the next, and so on.
The scientific article for the study is published in the renowned journal, Nature Communications: https://www.nature.com/ncomms/
The study's first author is René Schneider, affiliated with the University of Melbourne in Australia and the Max Planck Institute for Molecular Plant Physiology in Germany. In addition to the University of Copenhagen's Staffan Persson, research was also conducted by researchers from Wageningen University, Netherlands; Nara Institute of Science and Technology, Japan; University of Tasmania, Australia and Shanghai Jiao Tong University in China.
Professor Staffan Persson was recruited from the University of Melbourne, Australia in July 2020 as a recipient of the Villum Investigator and NNF Laureate Research Grant. Persson is the new head of the Copenhagen Plant Science Center (CPSC) and a DNRF Chair at the Department of Plant and Environmental Sciences.
Like these kind of feel good stories? Get the Brighter Side of News' newsletter.
Tags: #Green_Good_News, #Carbon_Emissions, #Trees, #Construction, #The_Brighter_Side_of_News
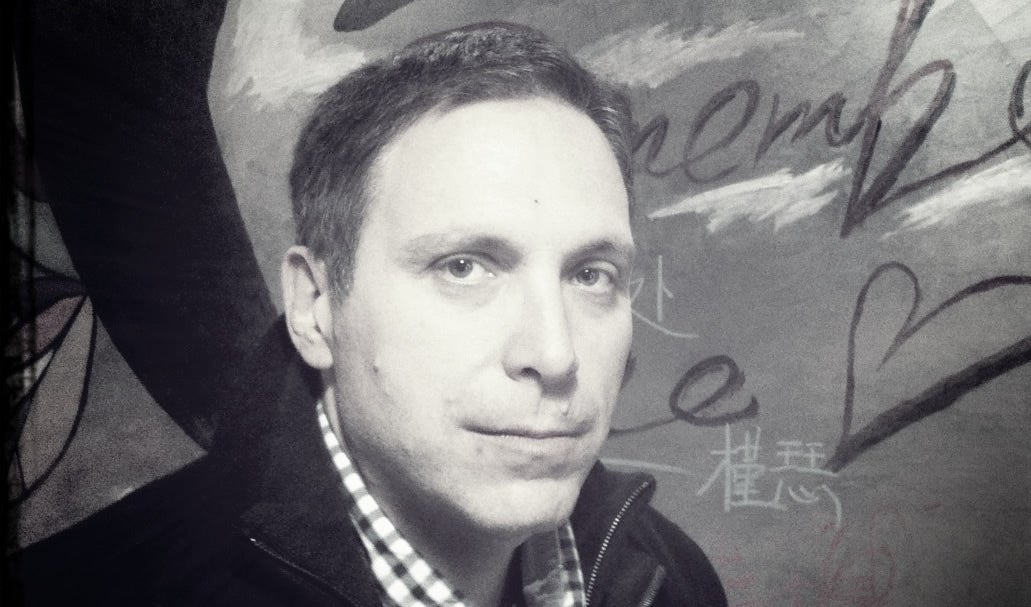
Joseph Shavit
Head Science News Writer | Communicating Innovation & Discovery
Based in Los Angeles, Joseph Shavit is an accomplished science journalist, head science news writer and co-founder at The Brighter Side of News, where he translates cutting-edge discoveries into compelling stories for a broad audience. With a strong background spanning science, business, product management, media leadership, and entrepreneurship, Joseph brings a unique perspective to science communication. His expertise allows him to uncover the intersection of technological advancements and market potential, shedding light on how groundbreaking research evolves into transformative products and industries.