Researchers develop a more efficient and cleaner approach to extracting rare earth elements
A new breakthrough in science could reshape how rare earth elements are separated, extracted, and reused—something that matters deeply in the race for green energy and high-tech manufacturing. These metallic…
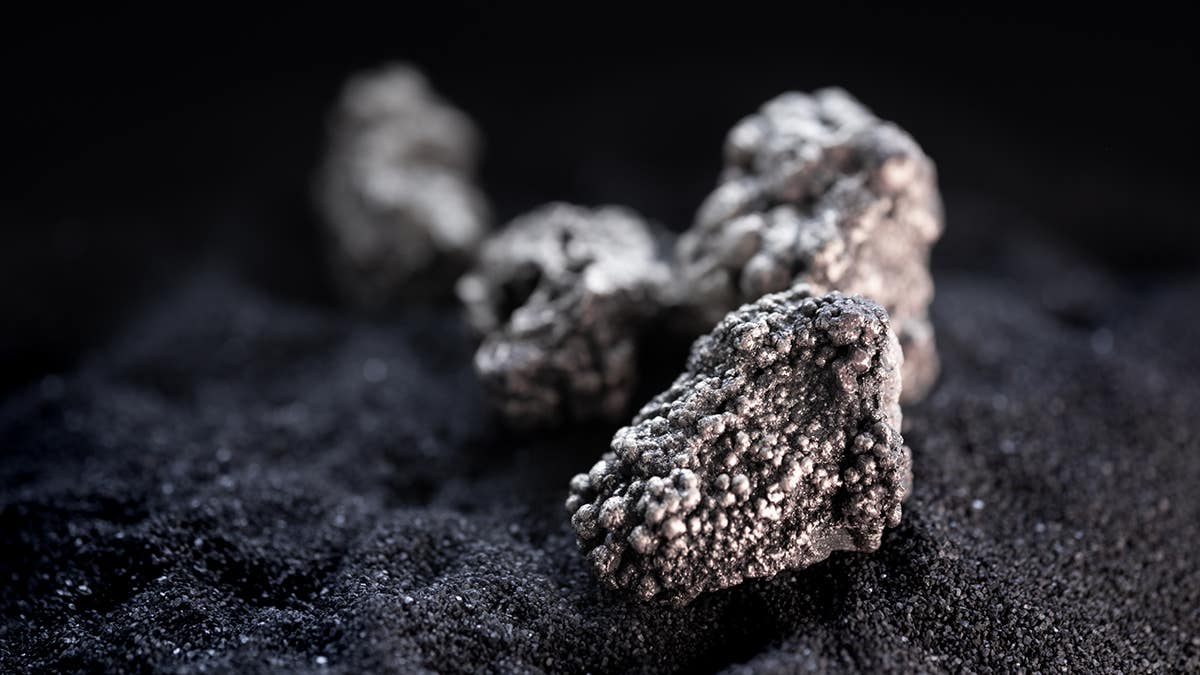
Artificial channels inspired by biology offer a cleaner, more precise way to extract rare earth elements. (CREDIT: Phawat, Adobe Stock)
A new breakthrough in science could reshape how rare earth elements are separated, extracted, and reused—something that matters deeply in the race for green energy and high-tech manufacturing. These metallic elements, vital for powering electric vehicles, smartphones, wind turbines, and energy-efficient lights, are notoriously difficult to isolate. But researchers at The University of Texas at Austin have created a new method that may change everything.
The Challenge With Rare Earth Elements
Rare earth elements, often called REEs, are a group of 17 metals including lanthanides, scandium, and yttrium. Despite the name, they're not especially rare in the Earth's crust. What makes them "rare" is how hard it is to separate them from each other. They have almost the same size and chemical properties. In water, their ionic radii differ by just about 0.02 nanometers.
Traditional separation methods rely on long, costly processes. These often use toxic solvents and large amounts of energy. Worse, they generate harmful waste. For instance, extracting europium and terbium—two middle rare earth elements—requires multiple chemical steps. Each step adds cost and pollution. These methods also struggle to recover REEs from recycled electronics and industrial waste.
Current demand for REEs, especially those critical to green technology, is rising fast. The U.S. Department of Energy and the European Commission have marked elements like europium and terbium as high risk due to their limited supply and rising use. Demand could jump over 2,600% by 2035. That creates an urgent need for cleaner, cheaper, and more efficient ways to get these materials.
A Nature-Inspired Solution
That’s where the team from Austin comes in. Drawing inspiration from how living cells transport ions, the researchers developed artificial membrane channels. These channels act like tiny gatekeepers. They let in specific ions and block the rest. In biology, this selectivity is key to brain signals and muscle movement. Now, it's being used to extract REEs.
The artificial channels are built using a structure called a pillararene. The scientists modified this ring-shaped molecule by attaching diphenylphosphine oxide (DPP) ligands. These long side chains help the channel recognize and transport specific ions. The result? A high-tech filter that allows only middle rare earth elements like europium (Eu³⁺) and terbium (Tb³⁺) to pass.
Related Stories
“Inspired by the natural world, we wanted to copy biology’s ability to move ions with extreme accuracy,” said Manish Kumar, professor at UT Austin’s Cockrell School of Engineering. Kumar has spent more than five years developing this system. His lab specializes in membrane technology, especially for clean water.
Record-Breaking Selectivity
The results are impressive. In lab tests, the artificial membrane channels showed over 40 times more selectivity for europium over lanthanum, a lighter rare earth element. The channels also favored europium over ytterbium, a heavier one, by a factor of 30. Terbium showed even stronger selectivity: 140 times more than lanthanum, 72 times more than ytterbium, and nearly 60 times more than neodymium.
For comparison, traditional solvent methods often achieve selectivity ratios of just 3 to 5 per step. That means dozens of steps are needed to isolate a single element. These new channels can do in one step what used to take many—saving time, money, and energy.
“Nature has perfected the art of selective transport through biological membranes,” said Venkat Ganesan, a chemical engineering professor involved in the research. “These artificial channels are like tiny gatekeepers, allowing only the desired ions to pass through.”
How It Works
So what gives these channels their edge? It comes down to water. Using molecular dynamics simulations, the team discovered that the channels rely on water-mediated interactions. When rare earth ions are in water, they are surrounded by eight or nine water molecules. The way each ion interacts with these waters varies slightly. These subtle differences are enough for the channel to tell them apart.
The DPP ligands inside the channel create a narrow space. Only ions with the right size and water shell fit. If the match isn’t perfect, the ion gets rejected. That kind of precision is difficult to achieve with solvents, even when using advanced chemical ligands.
The channels also block other common ions, including potassium, sodium, calcium, and magnesium. That adds another layer of selectivity and makes the method useful for recovering REEs from waste, where many different ions are present.
Beyond Rare Earths
Though this work focuses on europium and terbium, the team sees bigger potential. The same channel technology could be adapted to target other critical elements like lithium, cobalt, gallium, and nickel. These are also vital for batteries and electronics, and they face similar supply risks.
The artificial channels are being designed for use in scalable membrane systems. That means industries could use them continuously, rather than in batches like current ion-exchange methods. This change could streamline REE processing and reduce reliance on foreign suppliers, while cutting environmental costs.
“This is a first step towards translating nature’s sophisticated molecular recognition and transport strategies into robust industrial processes,” said Harekrushna Behera, a research associate in Kumar’s lab. “It brings high selectivity to settings where current methods fall short.”
A Greener Future
Scientists have explored other alternatives for REE separation, including ionic liquids and solid adsorbents like metal-organic frameworks (MOFs) and covalent organic frameworks (COFs). While these offer some advantages, they often use traditional chemical ligands. That limits how well they can tell similar elements apart.
By contrast, the biomimetic channels offer a fresh strategy. They rely on shape, water interactions, and molecular fit rather than just chemical affinity. This opens new doors for making separation technology more like biology—efficient, compact, and clean.
Kumar’s team hopes to build a platform that lets industries choose which ions to recover. That kind of customization could help address shortages across many sectors. As more electronics are built and more devices are thrown away, having better ways to reclaim these metals becomes not just helpful—but essential.
This work, published in ACS Nano, is supported by multiple departments at UT Austin, including the Fariborz Maseeh Department of Civil, Architectural and Environmental Engineering, the McKetta Department of Chemical Engineering, and the Department of Chemistry in the College of Natural Sciences. Contributors include Tyler J. Duncan, Laxmicharan Samineni, Hyeonji Oh, Ankit Jogdand, Arnav Karnik, Raman Dhiman, Aida Fica, and Tzu-Yun Hsieh.
If this technology moves beyond the lab and into factories, it could rewrite how rare earths are harvested—from mining to recycling—paving the way for a cleaner and more sustainable future.
Note: The article above provided above by The Brighter Side of News.
Like these kind of feel good stories? Get The Brighter Side of News' newsletter.
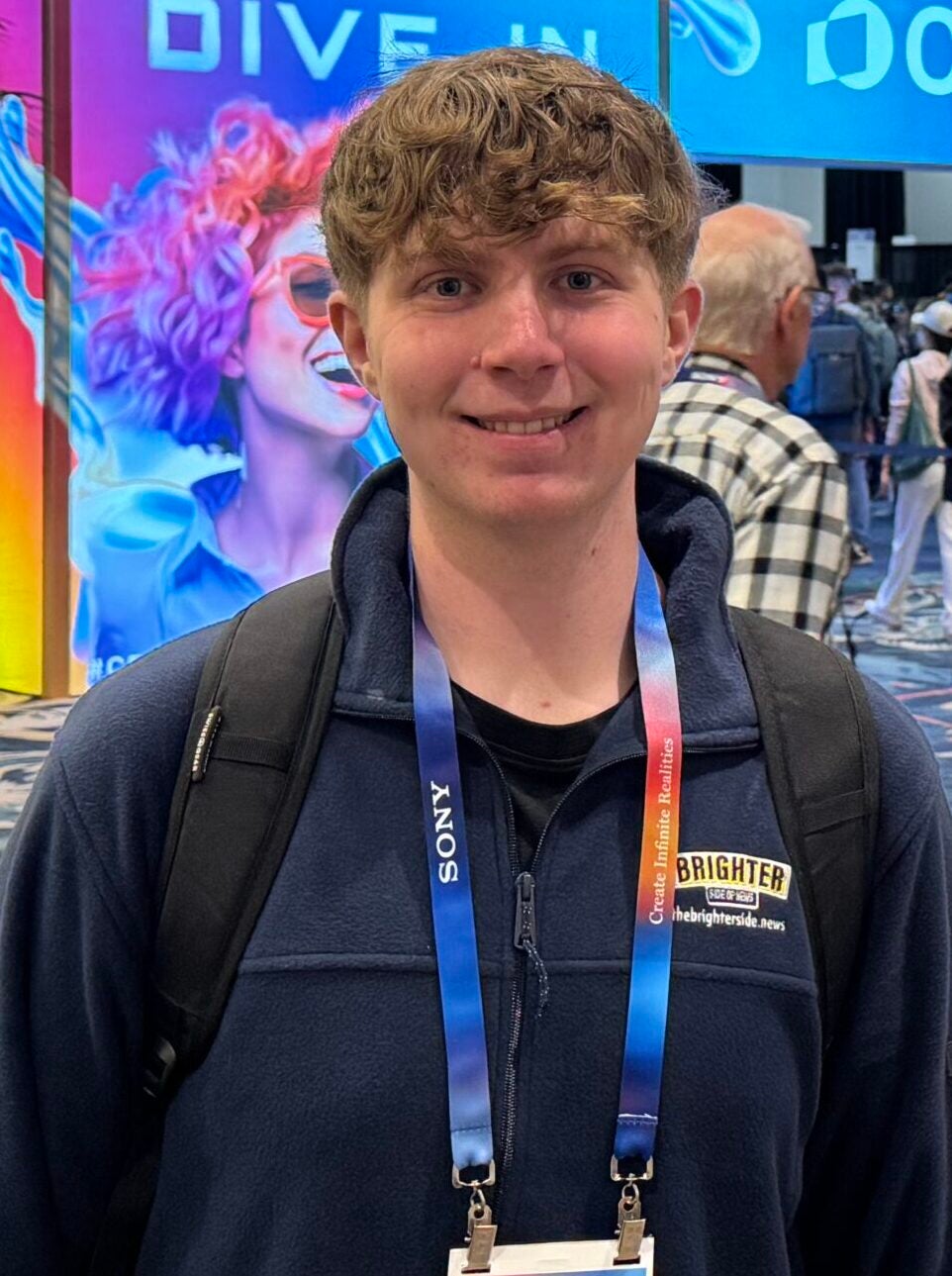
Joshua Shavit
Science & Technology Writer | AI and Robotics Reporter
Joshua Shavit is a Los Angeles-based science and technology writer with a passion for exploring the breakthroughs shaping the future. As a contributor to The Brighter Side of News, he focuses on positive and transformative advancements in AI, technology, physics, engineering, robotics and space science. Joshua is currently working towards a Bachelor of Science in Business Administration at the University of California, Berkeley. He combines his academic background with a talent for storytelling, making complex scientific discoveries engaging and accessible. His work highlights the innovators behind the ideas, bringing readers closer to the people driving progress.