Researchers enter the dawn of solid-state quantum networks
Since the early days of table top experiments, one of the most significant challenges is to extend the distance of quantum communications.
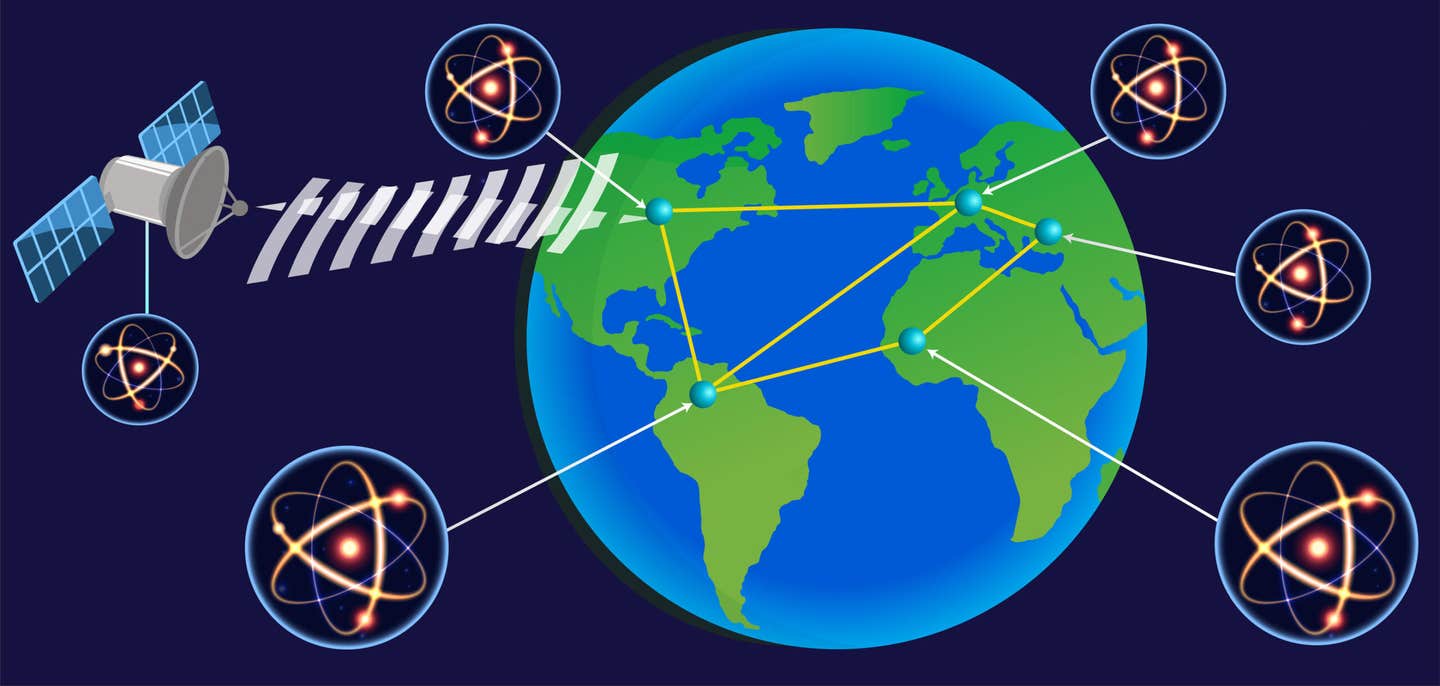
[Jan. 2, 2023: Daneet Steffens, SPIE--International Society for Optics and Photonics]
Schematic overview of a phase-separated Anderson localization fiber as quantum channel between a transmitter and receiver. The illustration shows that quantum correlations such as entanglement are maintained during transport from the transmitter (generation) to receiver (detection) all the way along the fiber. (CREDIT: ICFO/ A. Cuevas)
Quantum communications exploit the fundamental properties of quantum mechanics, such as superposition and entanglement, to implement communication tasks that are infeasible with classical means. Examples include quantum key distribution and quantum teleportation.
Since the early days of table top experiments, one of the most significant challenges of the field is to extend the distance of quantum communication to a practically useful scale. Exciting progress has been made over the past decades that culminated in satellite-based quantum communication over 1000 km. Taking advantage of the empty outer space, the satellite-based transmission channel showed a much lower loss than the optical fibers.
This year’s Nobel Prize in Physics celebrated the fundamental interest of quantum entanglement, and also envisioned the potential applications in “the second quantum revolution” — a new age when we are able to manipulate the weirdness of quantum mechanics, including quantum superposition and entanglement.
A large-scale and fully functional quantum network is the holy grail of quantum information sciences. It will open a new frontier of physics, with new possibilities for quantum computation, communication, and metrology.
Related Stories
One of the most significant challenges is to extend the distance of quantum communication to a practically useful scale.
Unlike classical signals that can be noiselessly amplified, quantum states in superposition cannot be amplified because they cannot be perfectly cloned. Therefore, a high-performance quantum network requires not only ultra-low-loss quantum channels and quantum memory, but also high-performance quantum light sources.
There has been exciting recent progress in satellite-based quantum communications and quantum repeaters, but a lack of suitable single-photon sources has hampered further advances.
What is required of a single-photon source for quantum network applications? First, it should emit one (only one) photon at a time. Second, to attain brightness, the single-photon sources should have high system efficiency and a high repetition rate. Third, for applications such as in quantum teleportation that require interfering with independent photons, the single photons should be indistinguishable.
Experimental configuration of quantum interference between two independent solid-state QD single-photon sources separated by 302 km fiber. DM: dichromatic mirror, LP: long pass, BP: band pass, BS: beam splitter, SNSPD: superconducting nanowire single- photon detector, HWP: half-wave plate, QWP: quarter-wave plate, PBS: polarization beam splitter. (CREDIT: Advanced Photonics)
Additional requirements include a scalable platform, tunable and narrowband linewidth (favorable for temporal synchronization), and interconnectivity with matter qubits.
A promising source is quantum dots (QDs), semiconductor particles of just a few nanometers. However, in the past two decades, the visibility of quantum interference between independent QDs has rarely exceeded the classical limit of 50% and distances have been limited to around a few meters or kilometers.
Quantum interference between two solid-state QD single photon sources. (a)–(d) Measurements of coincidence counts between two downconverted photons separated by total fiber lengths of 24 m, 101 km, 201 km, and 302 km, respectively (the 24 m is from the photon collection system before QFC). (CREDIT: Advanced Photonics)
As reported in Advanced Photonics, an international team of researchers has achieved high-visibility quantum interference between two independent QDs linked with ~300 km optical fibers.
They report efficient and indistinguishable single-photon sources with ultra-low-noise, tunable single-photon frequency conversion, and low-dispersion long fiber transmission. The single photons are generated from resonantly driven single QDs deterministically coupled to microcavities.
Characterization of single photons emitted from QD1 and QD2, respectively. (a) Single photon purity, HBT measurements give gQD1(2)(0)=0.072(1) and gQD2(2)(0)=0.051(1). (CREDIT: Advanced Photonics)
Quantum frequency conversions are used to eliminate the QD inhomogeneity and shift the emission wavelength to the telecommunications band. The observed interference visibility is up to 93%.
According to senior author Chao-Yang Lu, professor at the University of Science and Technology of China (USTC), “Feasible improvements can further extend the distance to ~600 km.”
Lu remarks, “Our work jumped from the previous QD-based quantum experiments at a scale from ~1 km to 300 km, two orders of magnitude larger, and thus opens an exciting prospect of solid-state quantum networks.” With this reported jump, the dawn of solid-state quantum networks may soon begin breaking toward day.
For more science and technology news stories check out our New Innovations section at The Brighter Side of News.
Note: Materials provided above by SPIE--International Society for Optics and Photonics. Content may be edited for style and length.
Like these kind of feel good stories? Get the Brighter Side of News' newsletter.
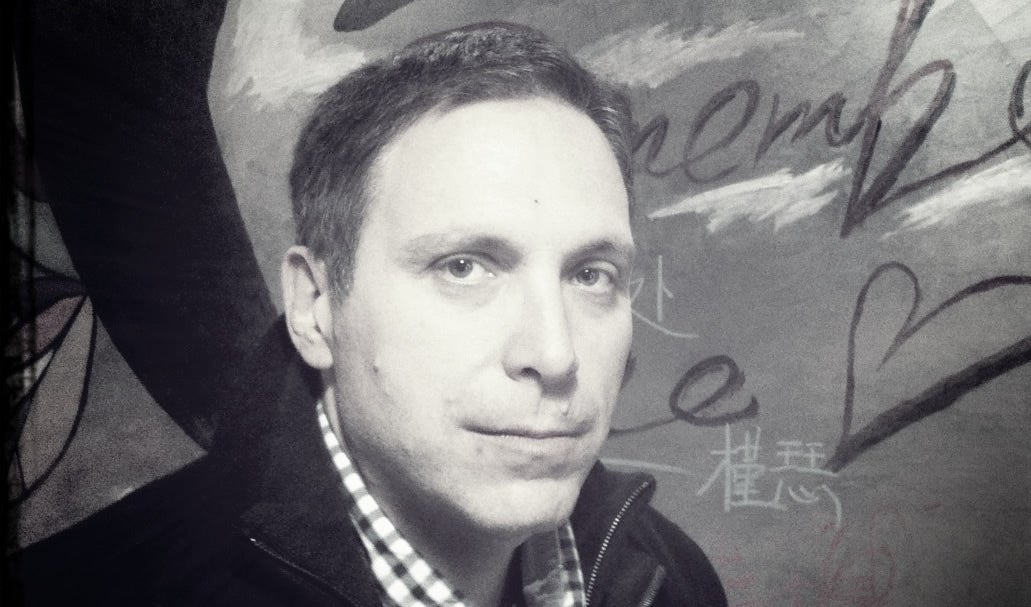
Joseph Shavit
Head Science News Writer | Communicating Innovation & Discovery
Based in Los Angeles, Joseph Shavit is an accomplished science journalist, head science news writer and co-founder at The Brighter Side of News, where he translates cutting-edge discoveries into compelling stories for a broad audience. With a strong background spanning science, business, product management, media leadership, and entrepreneurship, Joseph brings a unique perspective to science communication. His expertise allows him to uncover the intersection of technological advancements and market potential, shedding light on how groundbreaking research evolves into transformative products and industries.