Researchers turn seawater into drinkable water and a renewable energy source
Researchers managed to increase the RFD system’s salt removal rate by approximately 20 percent while also reducing its energy demands
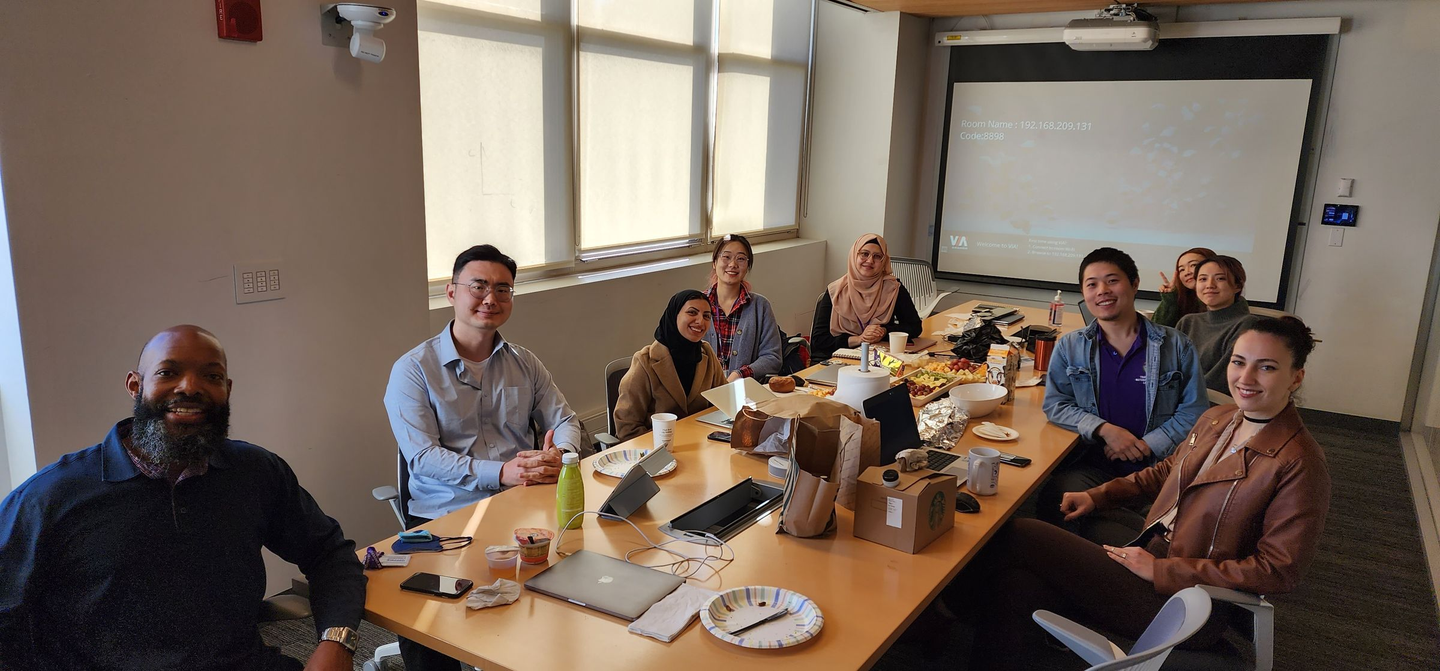
The NYU Tandon team, led by Dr. André Taylor, professor of chemical and biomolecular engineering and director of DC-MUSE . (CREDIT: NYU)
Researchers at NYU Tandon School of Engineering have achieved a significant breakthrough in the field of Redox Flow Desalination (RFD), an emerging electrochemical technique that has the potential to transform seawater into potable drinking water while also offering an affordable means of storing renewable energy.
In a recently published paper in Cell Reports Physical Science, the NYU Tandon team, led by Dr. André Taylor, professor of chemical and biomolecular engineering and director of DC-MUSE (Decarbonizing Chemical Manufacturing Using Sustainable Electrification), managed to increase the RFD system's salt removal rate by approximately 20 percent while simultaneously reducing its energy demand by optimizing fluid flow rates.
The implications of this achievement are substantial. RFD systems present a scalable and flexible solution for energy storage, enabling the efficient utilization of intermittent renewable energy sources like solar and wind. Beyond that, RFD offers a promising solution to the global water crisis.
Dr. Taylor envisions a future where energy storage and desalination are seamlessly integrated, creating a sustainable and efficient solution to meet the growing demand for freshwater, champion environmental conservation, and promote renewable energy integration.
Related Stories
This approach can not only reduce reliance on conventional power grids but also contribute to the transition toward a carbon-neutral and eco-friendly water desalination process. Furthermore, the integration of redox flow batteries with desalination technologies enhances system efficiency and reliability.
The success of this groundbreaking project is credited to Stephen Akwei Maclean, the paper's first author and a NYU Tandon Ph.D. candidate in chemical and biomolecular engineering. Maclean's exceptional skills in designing the system architecture using advanced 3D printing technology available at the NYU Maker Space played a pivotal role in this achievement.
The RFD system operates by dividing incoming seawater into two streams: the salinating stream and the desalinating stream, effectively separated by either a cation exchange membrane (CEM) or an anion exchange membrane (AEM).
Schematic for a four-channel RFD in single-pass mode with an A/A*, representing electrochemical reactions of redox species dissolved in conducting salts solutions, and channels separated by cation-exchange membrane (CEM) and anion-exchange membrane (AEM). (CREDIT: Cell Reports Physical Science)
Electrons are supplied from the cathode to the redox molecule, extracting Na+ that diffuses from the desalinating stream. The redox molecule and Na+ are then transported to another channel, where electrons are supplied to the anode from the redox molecules, and Na+ is allowed to diffuse into the salinating stream. This process results in the generation of freshwater.
Maclean explains, "We can control the incoming seawater residence time to produce drinkable water by operating the system in a single pass or batch mode."
Graphical Abstract: Increasing the flow rates of electrolyte channels reduces electrolyte-membrane interfacial resistances, promoting ionic fluxes across ion-exchange membranes. (CREDIT: Cell Reports Physical Science)
Moreover, in reverse operation, where the brine and freshwater are mixed, the stored chemical energy can be converted into renewable electricity. This unique capability positions RFD systems as a form of "battery" capable of capturing excess energy stored from solar and wind sources and releasing it on demand, providing a versatile and sustainable supplement to other electricity sources.
While further research is needed, the findings from the NYU Tandon team offer a promising avenue toward a more cost-effective RFD process. This advancement is particularly critical in addressing the global challenge of ensuring access to potable water, especially as climate change and population growth intensify, leading to more regions grappling with water shortages.
The experimental cycling of the RFD system in (B) batch mode and (C) in single-pass mode, where the recycling of salt channels into large reservoirs ensures a continuous influent supply at constant salinity. (CREDIT: Cell Reports Physical Science)
This research aligns perfectly with the mission of DC-MUSE, a collaborative initiative established at NYU Tandon dedicated to advancing research activities that reduce the environmental impact of chemical processes through the use of renewable energy. Dr. Taylor's work in renewable energy and sustainable energy storage during off-peak hours forms the foundation for this study.
In addition to Dr. Taylor and Maclean, a dedicated team of NYU Tandon researchers contributed to this study, including Syed Raza, Hang Wang, Chiamaka Igbomezie, Jamin Liu, Nathan Makowski, Yuanyuan Ma, Yaxin Shen, and Jason A. Röhrl. Collaborating across borders, Guo-Ming Weng from Shanghai Jiao Tong University in China also played a crucial role as a team member.
This publication is a significant milestone, marking the 100th publication from Dr. Taylor's Transformative Materials & Devices Lab, which was originally established at Yale University in 2008 and subsequently relocated to NYU Tandon in 2018. The lab focuses on developing innovative materials and devices for energy conversion and storage, reflecting Dr. Taylor's enduring commitment to transformative research in the field.
For more green news stories check out our Green Impact section at The Brighter Side of News.
Note: Materials provided above by Derek Markham. Content may be edited for style and length.
Like these kind of feel good stories? Get the Brighter Side of News' newsletter.

Joshua Shavit
Science & Technology Writer | AI and Robotics Reporter
Joshua Shavit is a Los Angeles-based science and technology writer with a passion for exploring the breakthroughs shaping the future. As a contributor to The Brighter Side of News, he focuses on positive and transformative advancements in AI, technology, physics, engineering, robotics and space science. Joshua is currently working towards a Bachelor of Science in Business Administration at the University of California, Berkeley. He combines his academic background with a talent for storytelling, making complex scientific discoveries engaging and accessible. His work highlights the innovators behind the ideas, bringing readers closer to the people driving progress.