Scientists revolutionize nuclear fusion reactors using ordinary magnets
The “MUSE” device, utilizes permanent magnets – the kind found on refrigerators – to confine plasma for potential fusion reactions.
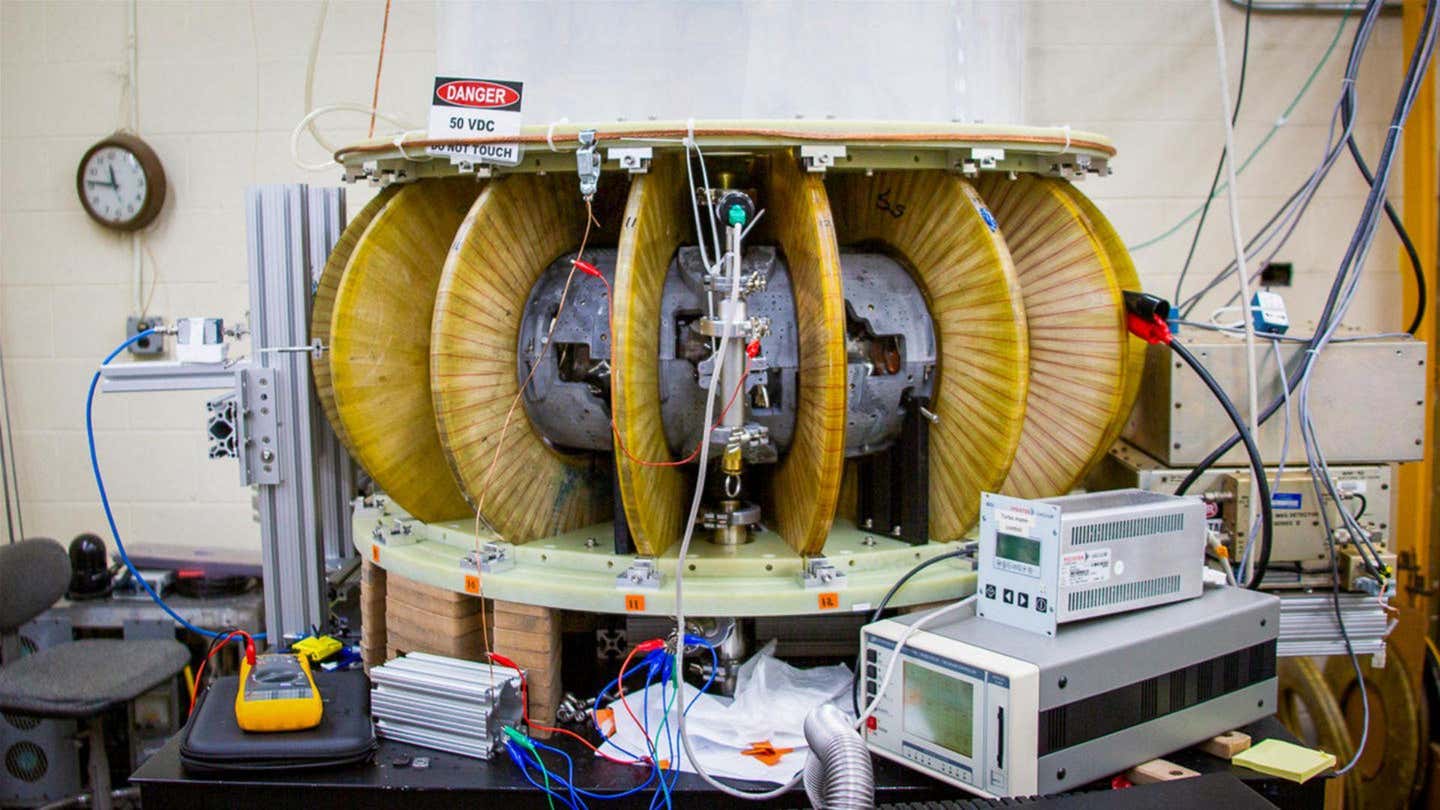
MUSE, the first stellarator built at PPPL in 50 years and the first ever to use permanent magnets. (CREDIT: Michael Livingston / PPPL Communications Department)
Scientists at the U.S. Department of Energy’s (DOE) Princeton Plasma Physics Laboratory (PPPL) have achieved a significant breakthrough in fusion energy research.
Their innovative design, the "MUSE" device, utilizes permanent magnets – the kind found on refrigerators – to confine plasma for potential fusion reactions. This surprisingly simple approach could dramatically reduce the cost of future fusion power plants.
Stellarators: Confining the Sun on Earth
Fusion – the process that powers stars like our Sun – offers a near-limitless source of clean energy. To achieve this on Earth, scientists rely on devices called stellarators and tokamaks to confine superheated plasma, the fuel for fusion reactions.
PPPL senior research physicist Michael Zarnstorff, left, stands next to plasma physics graduate student Tony Qian, right, with MUSE. (CREDIT: Tony Qian / PPPL)
Both machines utilize magnetic fields to contain the incredibly hot plasma. However, their methods differ. Tokamaks employ a strong electrical current flowing through the plasma itself, along with external coils, to generate the magnetic field. Stellarators, on the other hand, rely solely on intricate, twisted coils to create the necessary field. This makes stellarators inherently more stable and suitable for continuous operation, unlike tokamaks which experience current-driven instabilities.
While stellarators hold promise for future fusion power plants, their traditional Achilles' heel has been the complex and expensive electromagnets required to generate the magnetic field.
The PPPL team's MUSE device breaks away from this traditional approach. Instead of electromagnets, it utilizes readily available permanent magnets. This significantly simplifies construction and reduces costs associated with complex electromagnet design and operation.
Related Stories
"Using permanent magnets is a completely new way to design stellarators," explains Tony Qian, a graduate student whose research played a key role in MUSE's development. "This technique allows us to quickly test new plasma confinement ideas and build new devices with greater ease."
The concept of using permanent magnets in stellarators wasn't entirely new. However, translating theory into reality remained elusive for decades.
Senior research physicist Michael Zarnstorff at PPPL recognized this potential in 2014: "I realized that permanent magnets could generate and maintain the magnetic fields necessary to confine plasma for fusion reactions," he reveals.
Stellarators offer a key advantage over tokamaks – the issue of plasma stability. The electric currents used in tokamaks can introduce instabilities, making it difficult to sustain fusion reactions. Stellarators, lacking these currents, can operate continuously.
However, the intricate design and fabrication challenges associated with traditional stellarator electromagnets have hampered their progress. MUSE, with its readily available and easily shaped permanent magnets, could revolutionize the field.
Beyond Simplicity: The Power of Quasisymmetry
MUSE's design incorporates a crucial theoretical concept called quasisymmetry. Though the stellarator itself may appear irregular in shape, the strength of its magnetic field remains remarkably consistent throughout.
(LEFT) Some of the permanent magnets that make MUSE’s innovative concept possible. (RIGHT) A close-up of MUSE’s 3D-printed shell. (CREDIT: Xu Chu / PPPL and Michael Livingston / PPPL Communications Department)
This uniformity is essential for efficient plasma confinement, which is critical for achieving fusion. MUSE is designed to be exceptionally quasisymmetrical, surpassing previous stellarator designs.
The PPPL team is currently gearing up for experiments to assess MUSE's quasisymmetry and its impact on plasma confinement. The success of MUSE paves the way for a future where fusion power plants are more affordable and accessible, with permanent magnets playing a central role in this clean energy revolution.
PPPL founder Lyman Spitzer standing in front of the Model A stellarator, his invention and the first stellarator built at PPPL. (CREDIT: PPPL Archives)
The development of MUSE marks a significant milestone in the pursuit of clean and sustainable fusion energy. By utilizing readily available permanent magnets and achieving exceptional quasisymmetry, MUSE offers a simpler and potentially cheaper path towards achieving commercially viable fusion power. The coming experiments will be crucial in validating MUSE's capabilities and solidifying its role in the future of clean energy.
Types of Fusion Reactors
There are several types of fusion reactors under development worldwide. Here are some of the prominent ones:
Tokamak Reactors:
Examples: ITER (International Thermonuclear Experimental Reactor), JET (Joint European Torus), EAST (Experimental Advanced Superconducting Tokamak)
Tokamaks use magnetic confinement to contain the hot plasma, aiming to achieve conditions where fusion reactions can occur.
ITER, currently under construction in France, is the largest and most advanced tokamak project. It aims to demonstrate the feasibility of fusion power on a large scale.
While ITER is not expected to generate electricity for the grid, it aims to produce 500 megawatts of fusion power from an input of 50 megawatts of heating power (Q=10) during its experimental phase.
Stellarator Reactors:
Examples: Wendelstein 7-X (Germany), LHD (Large Helical Device) in Japan
Stellarators also use magnetic confinement like tokamaks but employ a different design to achieve the same goal of confining and heating the plasma.
Wendelstein 7-X is one of the most advanced stellarator projects. It aims to demonstrate continuous operation of a fusion reactor.
Inertial Confinement Fusion (ICF) Reactors:
Examples: National Ignition Facility (NIF) in the US, Laser Mégajoule (LMJ) in France
ICF reactors use high-energy lasers or particle beams to compress and heat a target containing fusion fuel (typically deuterium and tritium).
NIF aims to achieve ignition, where the fusion reactions produce more energy than the input energy from the lasers. However, as of the last update, ignition had not yet been achieved.
Magnetic Confinement Fusion (MCF) Reactors:
These are a broader category encompassing both tokamaks and stellarators, which use magnetic fields to confine the plasma.
The power outputs of experimental MCF reactors are typically measured in terms of fusion energy gain (Q), which represents the ratio of fusion power produced to the input power required to sustain the reaction. However, commercial-scale power production has not yet been achieved.
For more science news stories check out our New Innovations section at The Brighter Side of News.
Note: Materials provided above by The Brighter Side of News. Content may be edited for style and length.
Like these kind of feel good stories? Get the Brighter Side of News' newsletter.
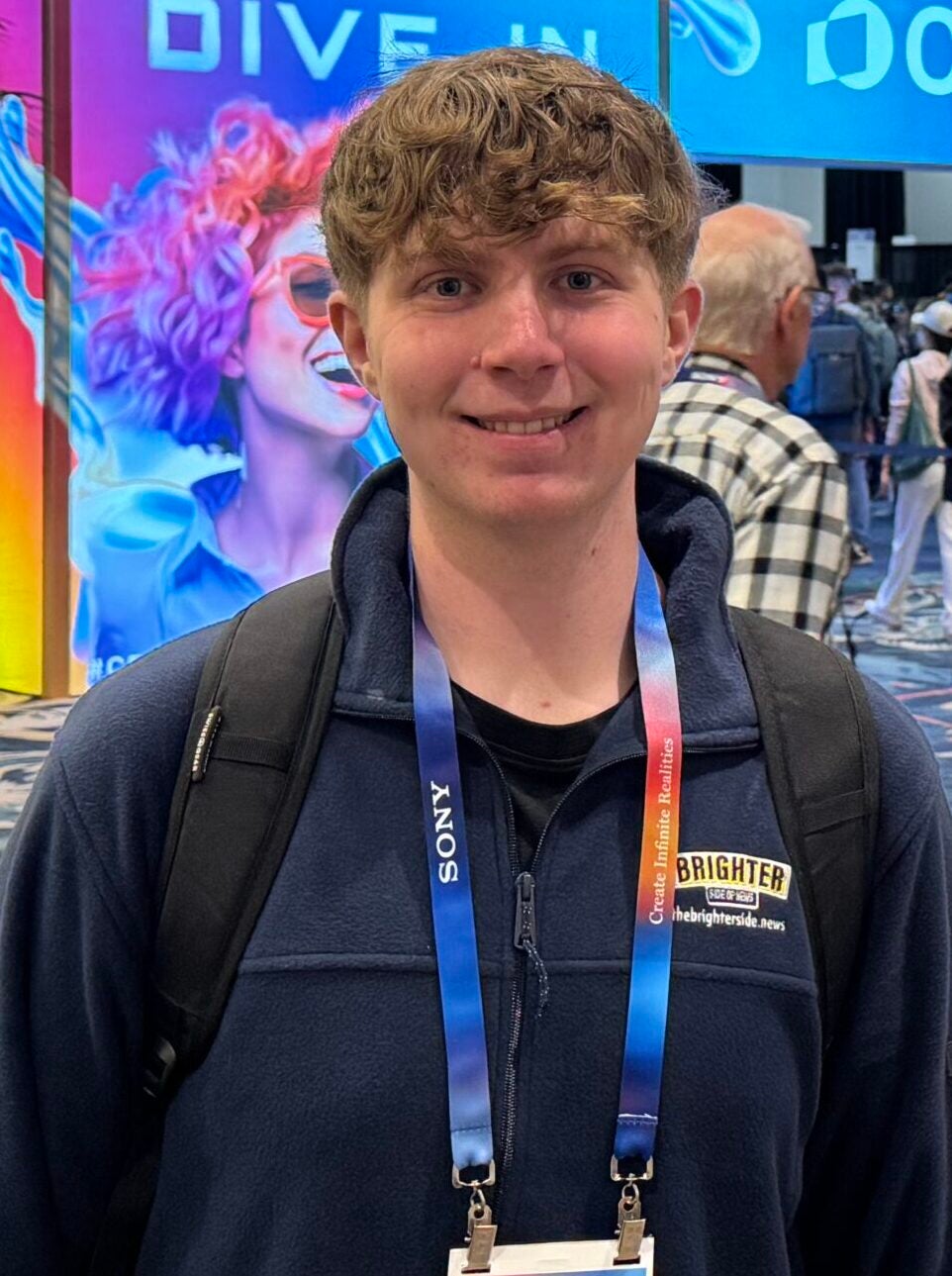
Joshua Shavit
Science & Technology Writer | AI and Robotics Reporter
Joshua Shavit is a Los Angeles-based science and technology writer with a passion for exploring the breakthroughs shaping the future. As a contributor to The Brighter Side of News, he focuses on positive and transformative advancements in AI, technology, physics, engineering, robotics and space science. Joshua is currently working towards a Bachelor of Science in Business Administration at the University of California, Berkeley. He combines his academic background with a talent for storytelling, making complex scientific discoveries engaging and accessible. His work highlights the innovators behind the ideas, bringing readers closer to the people driving progress.