Scientists unravel the mystery of the arrow of time
Study has made progress in identifying how particles and cells give rise to large-scale dynamics that we experience as the passage of time.
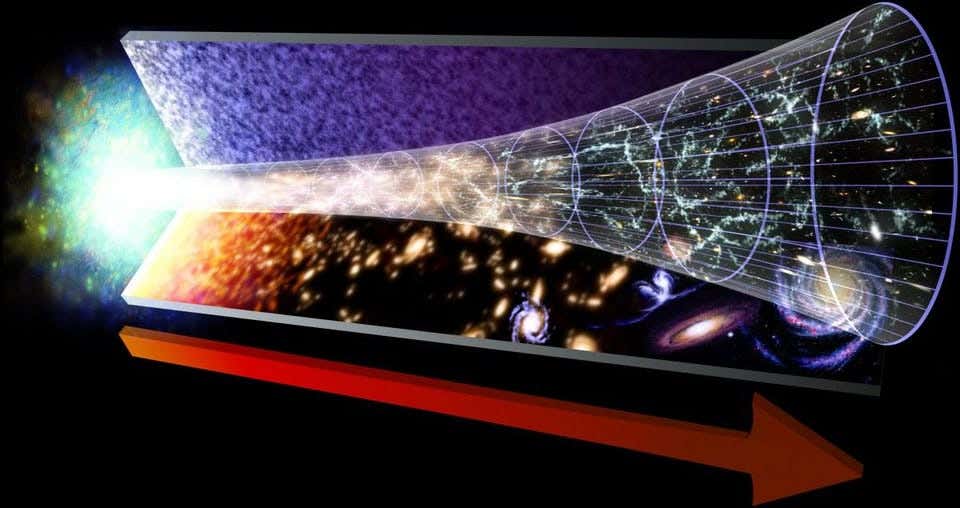
[Aug 23, 2022: Shawn Rhea, Advanced Science Research Center, GC/CUNY]
A new study has made progress toward identifying how particles and cells give rise to large-scale dynamics that we experience as the passage of time. (CREDIT: Creative Commons)
A new study from CUNY Graduate Center theoretical physicists has made progress toward identifying how particles and cells give rise to large-scale dynamics that we experience as the passage of time.
The flow of time from the past to the future is a central feature of how we experience the world. But precisely how this phenomenon, known as the arrow of time, arises from the microscopic interactions among particles and cells is a mystery—one that researchers at the CUNY Graduate Center Initiative for the Theoretical Sciences (ITS) are helping to unravel with the publication of a new paper in the journal Physical Review Letters. The findings could have important implications in a variety of disciplines, including physics, neuroscience, and biology.
Fundamentally, the arrow of time arises from the second law of thermodynamics: the principle that microscopic arrangements of physical systems tend to increase in randomness, moving from order to disorder. The more disordered a system becomes, the more difficult it is for it to find its way back to an ordered state, and the stronger the arrow of time. In short, the universe’s tendency toward disorder is the fundamental reason why we experience time flowing in one direction.
“The two questions our team had were, if we looked at a particular system, would we be able to quantify the strength of its arrow of time, and would we be able to sort out how it emerges from the micro scale, where cells and neurons interact, to the whole system?” said Christopher Lynn, the paper’s first author and a postdoctoral fellow with the ITS program. “Our findings provide the first step toward understanding how the arrow of time that we experience in daily life emerges from these more microscopic details.”
Related Stories
To begin answering these questions, the researchers explored how the arrow of time could be decomposed by observing specific parts of a system and the interactions between them. The parts, for example, could be the neurons that function within a retina. Looking at a single moment, they showed that the arrow of time can be broken down into different pieces: those produced by parts working individually, in pairs, in triplets or in more complicated configurations
Armed with this way of decomposing the arrow of time, the researchers analyzed existing experiments on the response of neurons in a salamander retina to different movies.
In one movie a single object moved randomly across the screen while another portrayed the full complexity of scenes found in nature. Across both movies, researchers found that the arrow of time emerged from the simple interactions between pairs of neurons—not large, complicated groups.
Local irreversibility in groups of neurons. (a-c) A group of three neurons in the salamander retina responding to a natural movie. (a) Points mark the times of action potentials in each of three neurons. To define neural states, we slide a window of width ∆t = 20 ms forward in time. When a cell generates an action potential inside the window we assign xi = 1, and when it is silent we assign xi = 0. Transitions occur when a spike enters the front or exits the back of the window, as shown. (b) The distribution P(x → x 0 ) over pairs of the eight possible states. Black entries indicate disallowed transitions. (c) Fluxes of probability P(x → x 0 ) − P(x 0 → x) between neural states, demonstrating that this group of neurons breaks detailed balance. (d) Distributions of local irreversibilities ˙I over 5-cell groups responding either to a natural movie (blue) or a Brownian stimulus (red), which is designed to be time-reversal invariant. Out of 100 random 5-cell groups, we only plot those (∼ 2/3) with significant local irreversibility. (CREDIT: Decomposing the local arrow of time in interacting systems)
Surprisingly, the team also observed that the retina showed a stronger arrow of time when watching random motion than a natural scene. Lynn said this latter finding raises questions about how our internal perception of the arrow of time becomes aligned with the external world.
These results may be of particular interest to neuroscience researchers,” said Lynn. “They could, for example, lead to answers about whether the arrow of time functions differently in brains that are neuroatypical.”
“Chris’ decomposition of local irreversibility—also known as the arrow of time—is an elegant, general framework that may provide a novel perspective for exploring many high-dimensional, nonequilibrium systems,” said David Schwab, a professor of Physics and Biology at the Graduate Center and the study’s principal investigator.
Authors in order: Christopher W. Lynn, Ph.D, postdoctoral fellow, CUNY Graduate Center; Caroline M. Holmes, Ph.D student, Princeton; William Bialek, Ph.D, Physics professor, CUNY Graduate Center; and David J. Schwab, Ph.D., Physics and Biology professor, CUNY Graduate Center
Funding sources: National Science Foundation, National Institutes of Health, James S McDonnell Foundation, Simons Foundation, and Alfred P Sloan Foundation.
For more science stories check out our New Discoveries section at The Brighter Side of News.
Note: Materials provided above by Advanced Science Research Center, GC/CUNY. Content may be edited for style and length.
Like these kind of feel good stories? Get the Brighter Side of News' newsletter.
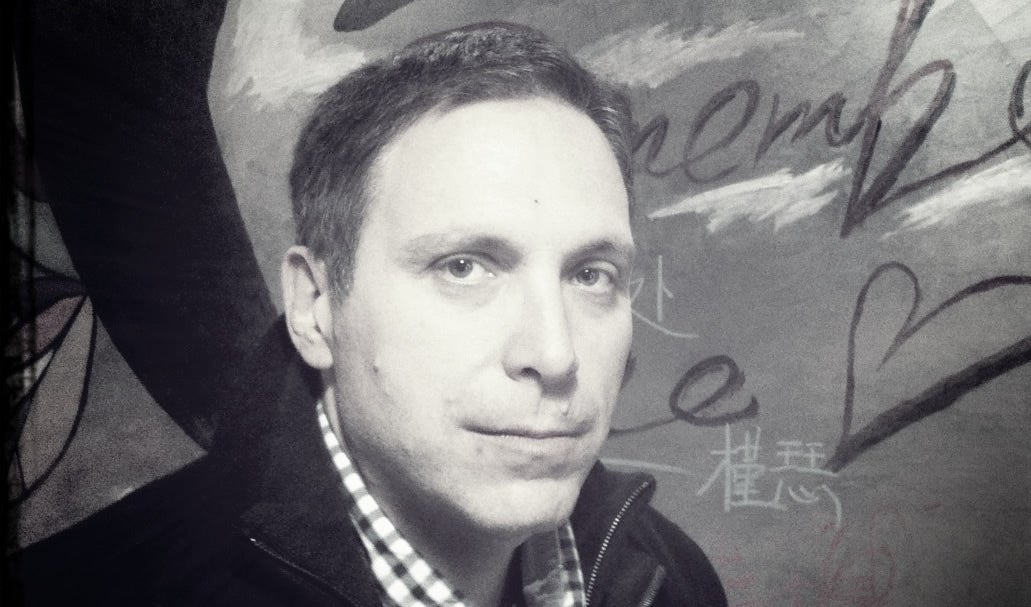
Joseph Shavit
Head Science News Writer | Communicating Innovation & Discovery
Based in Los Angeles, Joseph Shavit is an accomplished science journalist, head science news writer and co-founder at The Brighter Side of News, where he translates cutting-edge discoveries into compelling stories for a broad audience. With a strong background spanning science, business, product management, media leadership, and entrepreneurship, Joseph brings a unique perspective to science communication. His expertise allows him to uncover the intersection of technological advancements and market potential, shedding light on how groundbreaking research evolves into transformative products and industries.