Water extends much deeper into the Earth’s core than previously thought
In the vast, mysterious depths of our planet, a thin layer known as the E prime layer has intrigued seismologists for decades.
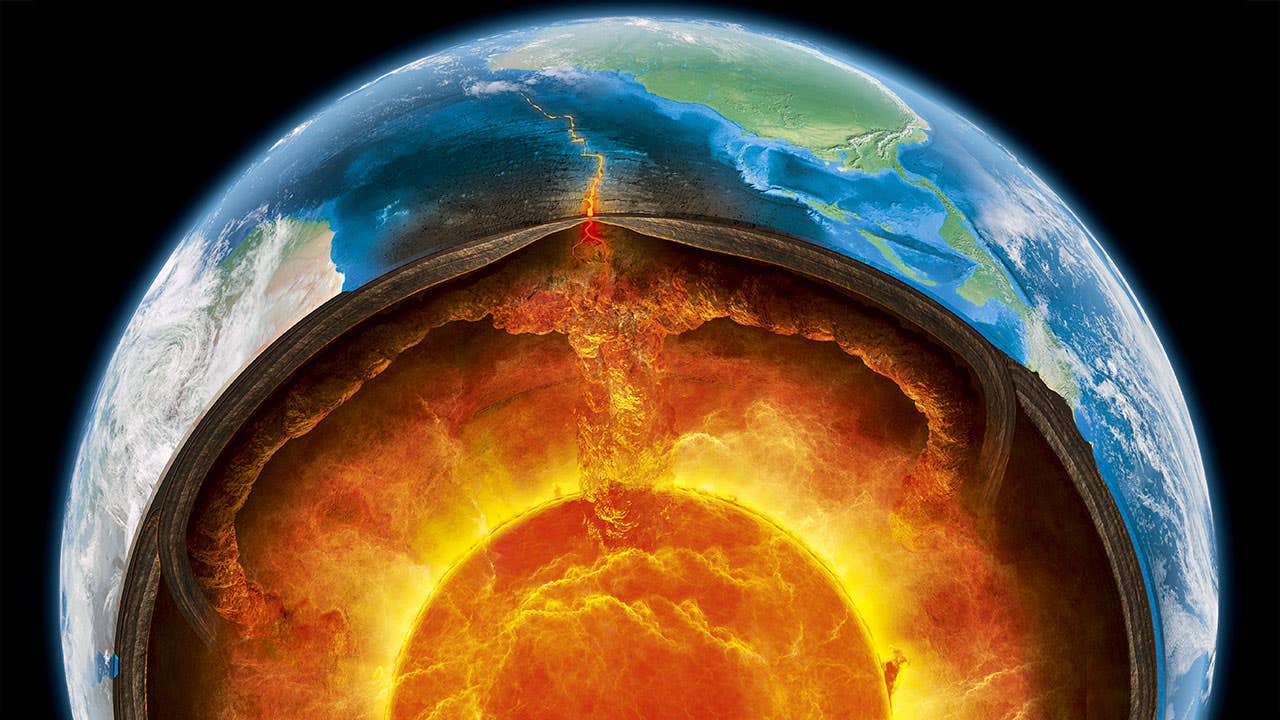
In this representation of the underground imaging, seismic waves from earthquakes in the southern hemisphere sample the ULVZ structure along the Earth’s core-mantle boundary and are recorded by sensors in Antarctica. (CREDIT: Drs. Edward Garnero and Mingming Li at Arizona State University)
In the vast depths of our planet, a thin layer known as the E prime layer has intrigued seismologists for decades. Just a few hundred kilometers thick, this mysterious layer's origin has long remained shrouded in mystery.
However, a recent breakthrough by an international team of researchers, including scientists from Arizona State University (ASU), has finally shed light on the origins of the E prime layer, revealing that Earth's surface water plays a crucial role in shaping the composition of our planet's metallic liquid core.
Published in the prestigious journal Nature Geoscience, this groundbreaking research has unveiled the intricate interplay between water from the Earth's surface and the deep regions of our planet, rewriting our understanding of Earth's internal processes and the extensive global water cycle.
Over the course of billions of years, water from the Earth's surface has been transported deep into the planet through the action of descending, or subducting, tectonic plates.
Related Stories
When this water reaches the core-mantle boundary, located a staggering 1,800 miles below the surface, it sets off a profound chemical reaction that fundamentally alters the core's structure.
Led by Dan Shim, Taehyun Kim, and Joseph O’Rourke from ASU's School of Earth and Space Exploration, in collaboration with Yong Jae Lee of Yonsei University in South Korea, the research team conducted high-pressure experiments to uncover the secrets of this transformation.
Their findings reveal that subducted water undergoes a chemical reaction with core materials, resulting in the formation of a hydrogen-rich, silicon-depleted layer. This layer effectively transforms the topmost outer core region into a film-like structure.
Illustration of silica crystals coming out from the liquid metal of the Earth's outer core due to a water-induced chemical reaction. (CREDIT: Dan Shim/ASU)
In addition to this structural alteration, the reaction generates silica crystals that rise and integrate into the mantle, further influencing the Earth's dynamics.
The implications of this discovery are far-reaching. The modified liquid metallic layer is expected to be less dense, with reduced seismic velocities. These characteristics align with the anomalous features that seismologists have previously observed and mapped, providing a compelling link between the research findings and real-world observations.
"For years, it has been believed that material exchange between Earth's core and mantle is small. Yet, our recent high-pressure experiments reveal a different story. We found that when water reaches the core-mantle boundary, it reacts with silicon in the core, forming silica," explained Dan Shim.
"This discovery, along with our previous observation of diamonds forming from water reacting with carbon in iron liquid under extreme pressure, points to a far more dynamic core-mantle interaction, suggesting substantial material exchange."
The revelation of this dynamic core-mantle interaction has profound implications for our understanding of Earth's internal processes. It suggests that the global water cycle is far more extensive than previously recognized, with the surface-water cycle intricately linked to the deep metallic core. This groundbreaking research challenges conventional thinking about the separation between the Earth's surface and its deep interior, highlighting the intricate connectivity of Earth's geophysical systems.
Proposed chemical exchange at the CMB and its effect on the evolution of the Earth’s topmost outer core. (CREDIT: Nature Geoscience)
To conduct this groundbreaking study, an international team of geoscientists employed advanced experimental techniques at two world-renowned facilities: the Advanced Photon Source of Argonne National Lab in the United States and PETRA III of Deutsches Elektronen-Synchrotron in Germany.
These cutting-edge facilities allowed the researchers to replicate the extreme conditions found at the core-mantle boundary, providing critical insights into the complex processes occurring deep within the Earth.
The pressure and temperature conditions of the reaction between Fe–Si alloys with different H2O sources, including hydrous silicate, hydrous aluminous silicate, Mg(OH)2 and H2O. (CREDIT: Nature Geoscience)
Key members of the research team from ASU included Taehyun Kim, who initiated the project as a visiting PhD student and is now a postdoctoral researcher at the School of Earth and Space Exploration.
Dan Shim, a professor at the School of Earth and Space Exploration, played a pivotal role in spearheading the high-pressure experimental work. Joseph O’Rourke, an assistant professor at the School of Earth and Space Exploration, leveraged computational simulations to gain a deeper understanding of the formation and persistence of the core's altered thin layer.
Yong Jae Lee led the research team from Yonsei University, and the project also benefited from the contributions of key research scientists Vitali Prakapenka and Stella Chariton at the Advanced Photon Source, as well as Rachel Husband, Nico Giordano, and Hanns-Peter Liermann at the Deutsches Elektronen-Synchrotron.
The discovery of the E prime layer's origins marks a significant milestone in our quest to understand the inner workings of our planet. Earth's core, once thought to be isolated from the surface, is now revealed as an intricately connected component of our planet's dynamic processes.
As we continue to unravel the mysteries of our world, this research reminds us of the profound interplay between Earth's surface and its deep interior, shaping our understanding of the planet we call home.
For more green news stories check out our Green Impact section at The Brighter Side of News.
Note: Materials provided above by The Brighter Side of News. Content may be edited for style and length.
Like these kind of feel good stories? Get the Brighter Side of News' newsletter.
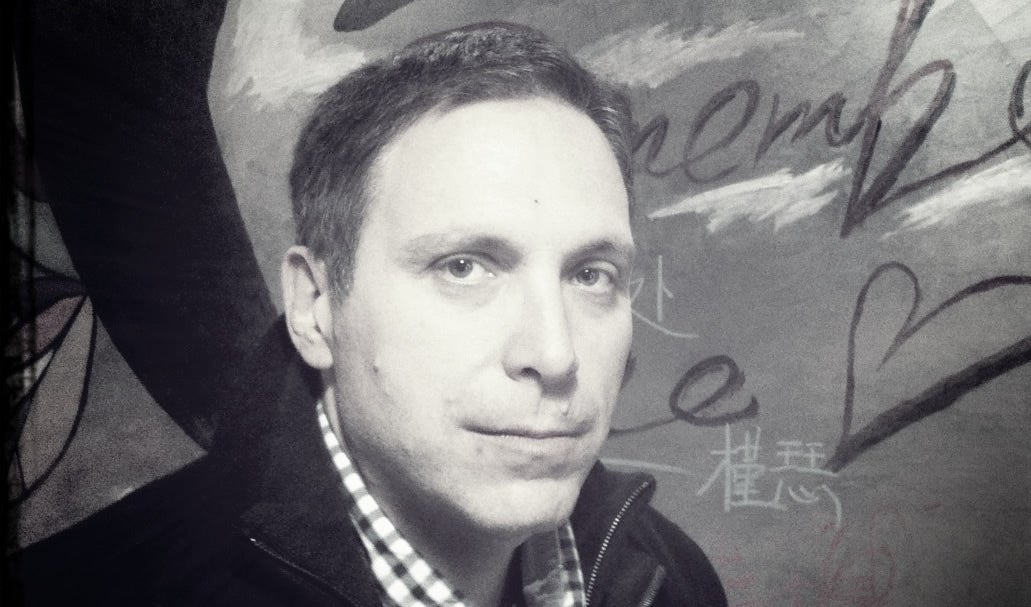
Joseph Shavit
Head Science News Writer | Communicating Innovation & Discovery
Based in Los Angeles, Joseph Shavit is an accomplished science journalist, head science news writer and co-founder at The Brighter Side of News, where he translates cutting-edge discoveries into compelling stories for a broad audience. With a strong background spanning science, business, product management, media leadership, and entrepreneurship, Joseph brings a unique perspective to science communication. His expertise allows him to uncover the intersection of technological advancements and market potential, shedding light on how groundbreaking research evolves into transformative products and industries.