Scientists discovered where black holes got their magnetic fields
Neutron stars pass magnetic fields to black holes, enabling powerful gamma-ray bursts in the universe’s most luminous explosions.
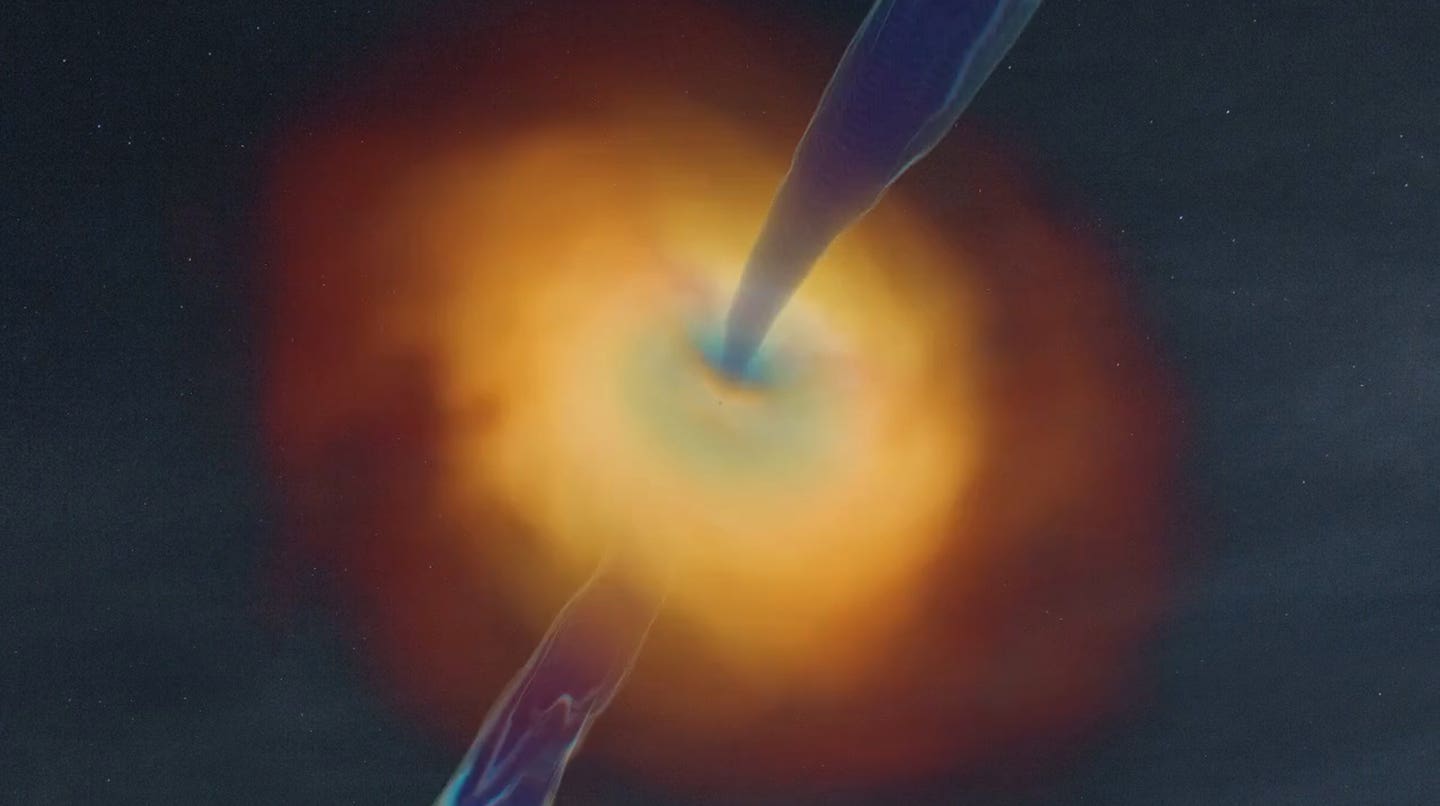
3D rendering of a rapidly spinning black hole’s accretion disk and a resulting black hole-powered jet. (CREDIT: Ore Gottlieb et al. (2024)
Black holes, often depicted as cosmic predators, have another fascinating role: they can launch some of the universe's most powerful explosions—gamma-ray bursts (GRBs). These bursts release more energy in a few seconds than our Sun will emit over its entire lifetime.
For decades, the origin of the intense magnetic fields driving these events has puzzled scientists. Recent findings from the Flatiron Institute and collaborators have provided a groundbreaking answer: black holes inherit their magnetic fields from their stellar ancestors—neutron stars.
When a massive star reaches the end of its life, it undergoes a dramatic supernova explosion. This leaves behind a compact remnant, typically a neutron star. Under certain conditions, this neutron star can further collapse into a black hole. This transformation, however, isn’t just about mass and gravity. It also involves the neutron star’s magnetic field.
According to Ore Gottlieb, a research fellow at the Flatiron Institute’s Center for Computational Astrophysics (CCA), “Proto-neutron stars are the mothers of black holes. When they collapse, a black hole is born, and it inherits the mother’s magnetic field.”
This inheritance allows black holes to retain powerful magnetic fields long enough to generate GRBs, solving a long-standing astrophysical puzzle.
Understanding Magnetic Field Paradoxes
For GRBs to occur, a black hole must meet two critical conditions: it must spin rapidly and possess a strong magnetic field. This combination allows the black hole to generate powerful jets of charged particles. However, these conditions seemed mutually exclusive.
A strong magnetic field in the progenitor star could slow its rotation, preventing the formation of an accretion disk. Without a disk, the black hole wouldn’t be able to power jets or produce GRBs. Conversely, a weak magnetic field wouldn’t provide the necessary energy to drive these jets.
Related Stories
“You need two things for jets to form: a strong magnetic field and an accretion disk,” says Gottlieb. “But high magnetism stops disk formation, and low magnetism can't produce jets. It appeared to be a paradox.”
The Flatiron team focused on solving this paradox by revisiting the role of accretion disks. Previous models primarily studied isolated neutron stars and black holes, where magnetic fields were assumed to dissipate during collapse.
However, the researchers discovered that collapsing neutron stars often retain accretion disks, which play a crucial role in preserving and transferring magnetic fields.
“Neutron stars with accretion disks can preserve their magnetic fields during collapse,” Gottlieb explains. “As the black hole forms, it inherits the magnetic field lines from the neutron star’s disk.”
Magnetic Field Amplification Mechanisms
Once a black hole inherits its magnetic field, it undergoes further amplification through various mechanisms within the accretion disk. These include:
- Magnetorotational Instability (MRI): This instability occurs in regions where angular velocity decreases outward, generating small-scale magnetic fields.
- Tayler-Spruit Dynamo: Active in stably stratified regions near the neutron star’s core, this mechanism amplifies toroidal magnetic fields.
- α−ω Dynamo: This dynamo operates in convective regions, producing large-scale dipolar magnetic fields, particularly in rapidly rotating systems.
Together, these mechanisms can amplify the magnetic field strength to as high as 1016 G10^{16} \, \text{G}1016G. This is more than enough to sustain the powerful jets required for GRBs.
Gamma-Ray Bursts: Cosmic Beacons
GRBs are among the brightest phenomena in the universe, observable across billions of light-years. These bursts occur when relativistic jets escape from the black hole’s poles, producing intense radiation in the gamma-ray spectrum.
The Flatiron Institute’s simulations demonstrate that the timescale for disk formation around a black hole is shorter than the timescale for magnetic field dissipation. This ensures that the black hole retains its inherited magnetic field long enough to produce a jet.
“Our models show that the black hole inherits its magnetic field from the neutron star before any significant dissipation occurs,” Gottlieb says. “This inheritance is critical for generating the jets that lead to GRBs.”
Black Hole Jets and the "No-Hair" Theorem
One challenge in understanding black hole magnetism lies in the "no-hair" theorem, which states that black holes cannot sustain magnetic fields independently. Any magnetic field they possess must come from external sources.
The study reveals that the inherited magnetic fields are pinned to the black hole’s accretion disk, delaying the dissipation process. However, without an ongoing external source, the black hole eventually loses its magnetism.
Simulations show that the loss of magnetic flux occurs over tens to hundreds of milliseconds—a brief window, but sufficient to power GRBs.
Implications for Stellar Evolution and Cosmology
This study has profound implications for understanding the life cycles of massive stars and their role in cosmic evolution. It redefines the conditions under which GRBs can form and highlights the importance of accretion disks in preserving magnetic fields.
“This discovery changes how we think about jet formation,” says Gottlieb. “Knowing that early disk formation implies magnetism allows us to reconsider the potential for GRB production in various stellar systems.”
The findings also open new avenues for exploring connections between different types of supernovae, neutron stars, and black holes.
The study, published in The Astrophysical Journal Letters, is the result of a multidisciplinary effort at CCA. Co-authors Brian Metzger, Jared Goldberg, Matteo Cantiello, and Mathieu Renzo contributed expertise in computational astrophysics, enabling high-fidelity simulations.
“This collaboration allowed us to address the problem from multiple angles,” Gottlieb says. “The computational resources at CCA were instrumental in running simulations that consistently captured the collapse process.”
Future Directions
Looking ahead, the team plans to refine their models to explore the variability in jet power and duration across different black hole systems. They aim to investigate how slight changes in the progenitor star’s magnetic field or rotation rate could impact the dynamics of GRB production.
“This is just the beginning,” Gottlieb notes. “There’s much more to learn about the interplay between magnetic fields, accretion disks, and jet formation. These findings lay the groundwork for deeper exploration of the universe’s most powerful explosions.”
Note: Materials provided above by The Brighter Side of News. Content may be edited for style and length.
Like these kind of feel good stories? Get The Brighter Side of News' newsletter.

Joshua Shavit
Science & Technology Writer | AI and Robotics Reporter
Joshua Shavit is a Los Angeles-based science and technology writer with a passion for exploring the breakthroughs shaping the future. As a contributor to The Brighter Side of News, he focuses on positive and transformative advancements in AI, technology, physics, engineering, robotics and space science. Joshua is currently working towards a Bachelor of Science in Business Administration at the University of California, Berkeley. He combines his academic background with a talent for storytelling, making complex scientific discoveries engaging and accessible. His work highlights the innovators behind the ideas, bringing readers closer to the people driving progress.