Next-gen quantum thermometer revolutionizes how we measure heat
A new thermometer based on Rydberg atoms measures heat using quantum physics, promising unmatched accuracy without contact or calibration.
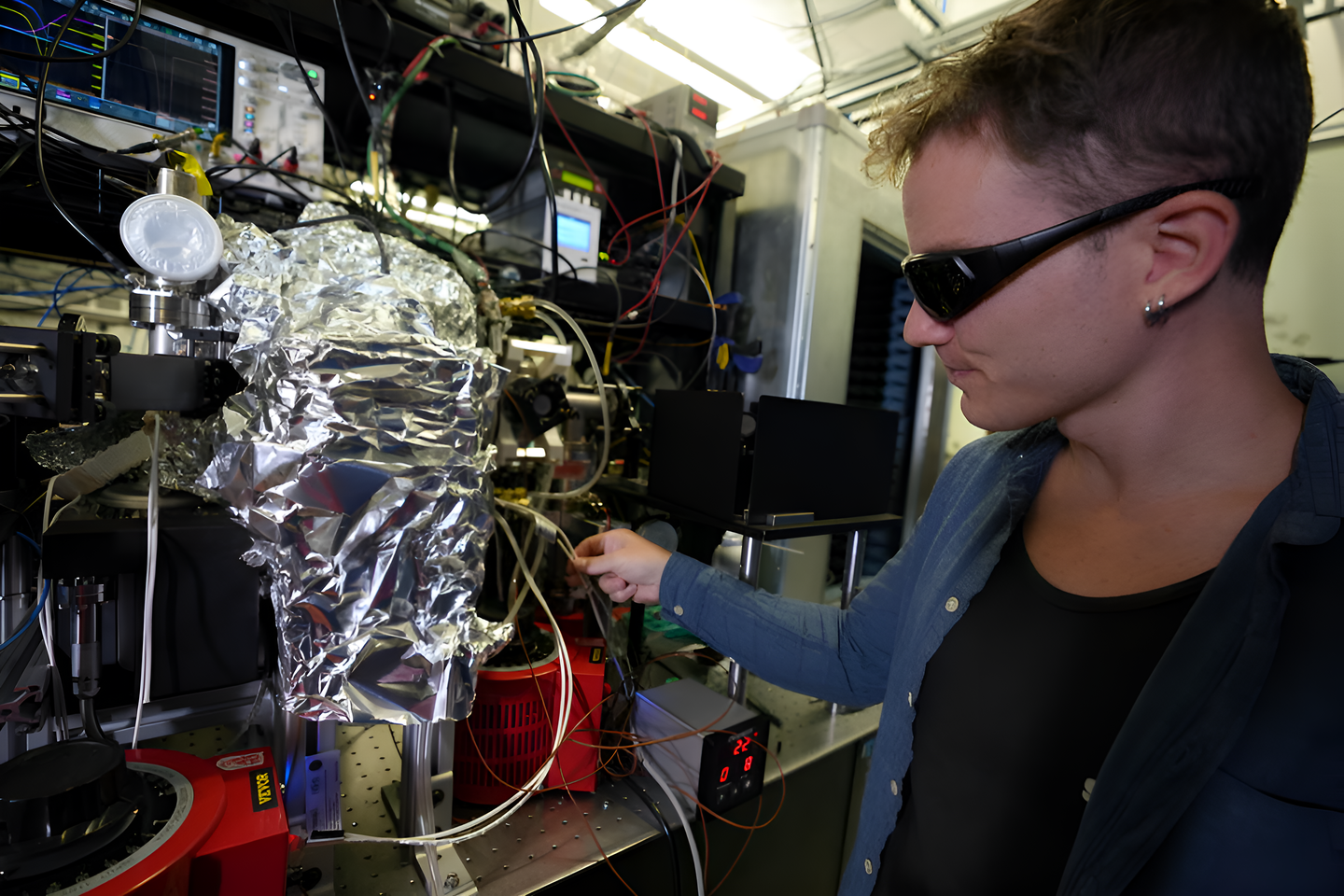
Scientists use Rydberg atoms to create a highly accurate thermometer that works without contact or calibration. (CREDIT: R. Jacobson)
Atoms are the tiny building blocks of all matter, but under the right conditions, they can become giants. Scientists have found a way to use these supersized atoms, called Rydberg atoms, to create a completely new kind of thermometer. Unlike a regular thermometer, this one doesn’t need to be touched or even adjusted. It works based on the laws of quantum physics—and it’s opening a new chapter in how we measure heat.
This new thermometer isn’t just another lab tool. It could improve everything from atomic clocks to advanced factories and space missions. By observing how these special atoms react to heat, scientists can now measure temperature with incredible accuracy, even without touching the object or needing to calibrate their device.
What Makes Rydberg Atoms So Special?
Atoms usually stay small and compact. But when hit with just the right energy, they expand—by a lot. In this case, researchers gave rubidium atoms a blast of laser energy. This forced their outermost electrons into very high orbits, far away from the nucleus. That turned these atoms into Rydberg atoms—about 1,000 times larger than usual.
In these inflated atoms, the outer electrons are extremely sensitive to their surroundings. This makes them perfect for detecting a specific kind of energy called blackbody radiation. That’s the invisible heat given off by everything around us. It increases as temperature rises, and Rydberg atoms feel that change more than normal atoms.
“When you boost an atom to a Rydberg state, it becomes like a giant antenna for heat,” said Noah Schlossberger, a postdoctoral researcher at the National Institute of Standards and Technology (NIST). “We’re essentially creating a thermometer that can provide accurate temperature readings without the usual calibrations that current thermometers require.”
How the Thermometer Works
To make their quantum thermometer, the NIST team started by placing rubidium gas in a vacuum chamber. They cooled it to near absolute zero—around 0.5 millikelvin—using lasers and magnetic fields. At this ultra-cold temperature, the atoms barely moved.
Related Stories
Then, lasers pushed the atoms’ electrons into far-off orbits, forming Rydberg atoms. Now, the researchers had a gas full of sensitive “quantum antennas” ready to measure heat.
As the environment warmed up, surrounding blackbody radiation caused the electrons in the Rydberg atoms to jump into even higher energy states. The rate of these jumps changed depending on the temperature. By tracking how many atoms changed states over time, the researchers could tell exactly how hot the environment was—no contact needed.
Using a method called selective field ionization, they identified which Rydberg states the atoms had entered. Their measurements focused on states with a principal quantum number around 30, which responded best to radiation near 130 gigahertz—just the right frequency range for the kind of heat emitted at room temperature.
The process also lined up closely with a theoretical model, proving the method's accuracy. “We reproduce the results with a simple semiclassical population transfer model,” one researcher said. That means the changes in energy levels followed expected physical rules, confirming the thermometer’s reliability.
Small Temperature Changes, Big Impact
This new thermometer can detect extremely small temperature changes. It showed a statistical sensitivity to fractional temperature uncertainty of 0.09 Hz⁻¹/². At room temperature, that’s like detecting changes as tiny as 26 Kelvin per square root of hertz. Even more impressively, the researchers calculated a systematic uncertainty of just 2 Kelvin—without any need for calibration.
That level of precision matters most in places where even tiny temperature shifts can throw off critical systems.
One of those systems is the atomic clock. These clocks depend on the behavior of atoms to keep time so precisely that they can measure the age of the universe to within a second. But even atomic clocks have a weakness—heat.
“Atomic clocks are exceptionally sensitive to temperature changes, which can cause small errors in their measurements,” said NIST research scientist Chris Holloway. “We’re hopeful this new technology could help make our atomic clocks even more accurate.”
Because Rydberg thermometers detect blackbody radiation directly and don’t require contact, they are perfect for monitoring temperature inside atomic clock chambers, where standard thermometers might not work well or could interfere.
A Quantum Tool for Real-World Needs
The Rydberg thermometer is more than a scientific curiosity. It has the potential to transform how we measure temperature in many places where traditional thermometers can’t go.
Factories that build microchips, for example, need precise temperature control during manufacturing. A tiny error could ruin an entire batch. In space, spacecraft experience wild temperature swings between sunlight and shade. Traditional thermometers struggle to perform in these extremes.
In both settings, a Rydberg thermometer could offer better reliability. It’s small, non-invasive, and doesn’t need regular calibration. Because it’s based on quantum principles, it works the same anywhere in the universe.
Scientists see even more possibilities ahead. The technology could support research in quantum computing, environmental science, and fundamental physics. Wherever accurate, contactless temperature readings are important, this new tool could play a role.
“This method opens a door to a world where temperature measurements are as reliable as the fundamental constants of nature,” said Holloway. “It’s an exciting step forward for quantum sensing technology.”
Why It Matters
What makes this breakthrough so meaningful is how it blends deep scientific theory with real-world usefulness. The technique works because Rydberg atoms react so strongly to electric fields and heat. Their response helps scientists read the “fingerprints” of temperature without any moving parts or outside adjustments.
In short, this is a thermometer built on nature’s own laws. It responds directly to the energy in the environment, translating that into a measurable signal. That signal is accurate, reliable, and repeatable.
With further development, this approach could change how people measure and manage heat across science and industry.
Researchers have proven that even the invisible forces in our world—like blackbody radiation—can become powerful tools when we understand how to read them. The Rydberg thermometer shows that the future of measurement may not lie in metal or glass, but in the strange and precise world of quantum physics.
Note: The article above provided above by The Brighter Side of News.
Like these kind of feel good stories? Get The Brighter Side of News' newsletter.
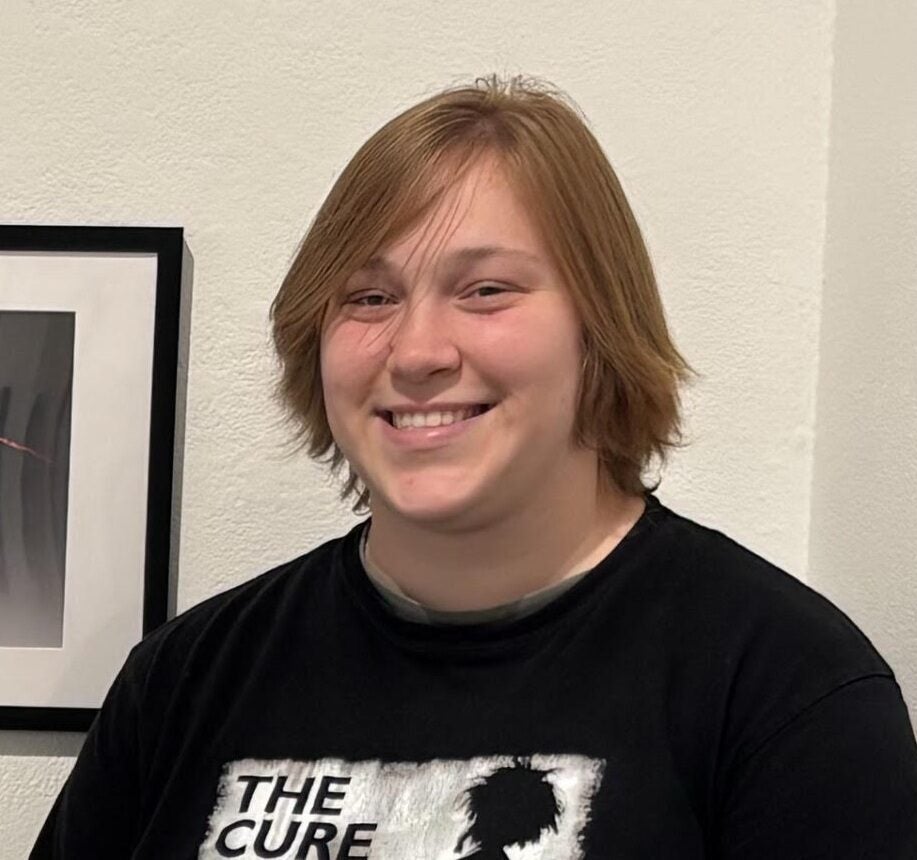
Mac Oliveau
Science & Technology Writer | AI and Robotics Reporter
Mac Oliveau is a Los Angeles–based science and technology journalist for The Brighter Side of News, an online publication focused on uplifting, transformative stories from around the globe. Passionate about spotlighting groundbreaking discoveries and innovations, Mac covers a broad spectrum of topics—from medical breakthroughs and artificial intelligence to green tech and archeology. With a talent for making complex science clear and compelling, they connect readers to the advancements shaping a brighter, more hopeful future.