Physicists rewrite quantum rules by bending light through both time and space
A team of physicists has reimagined one of science’s most iconic experiments—but in the dimension of time.
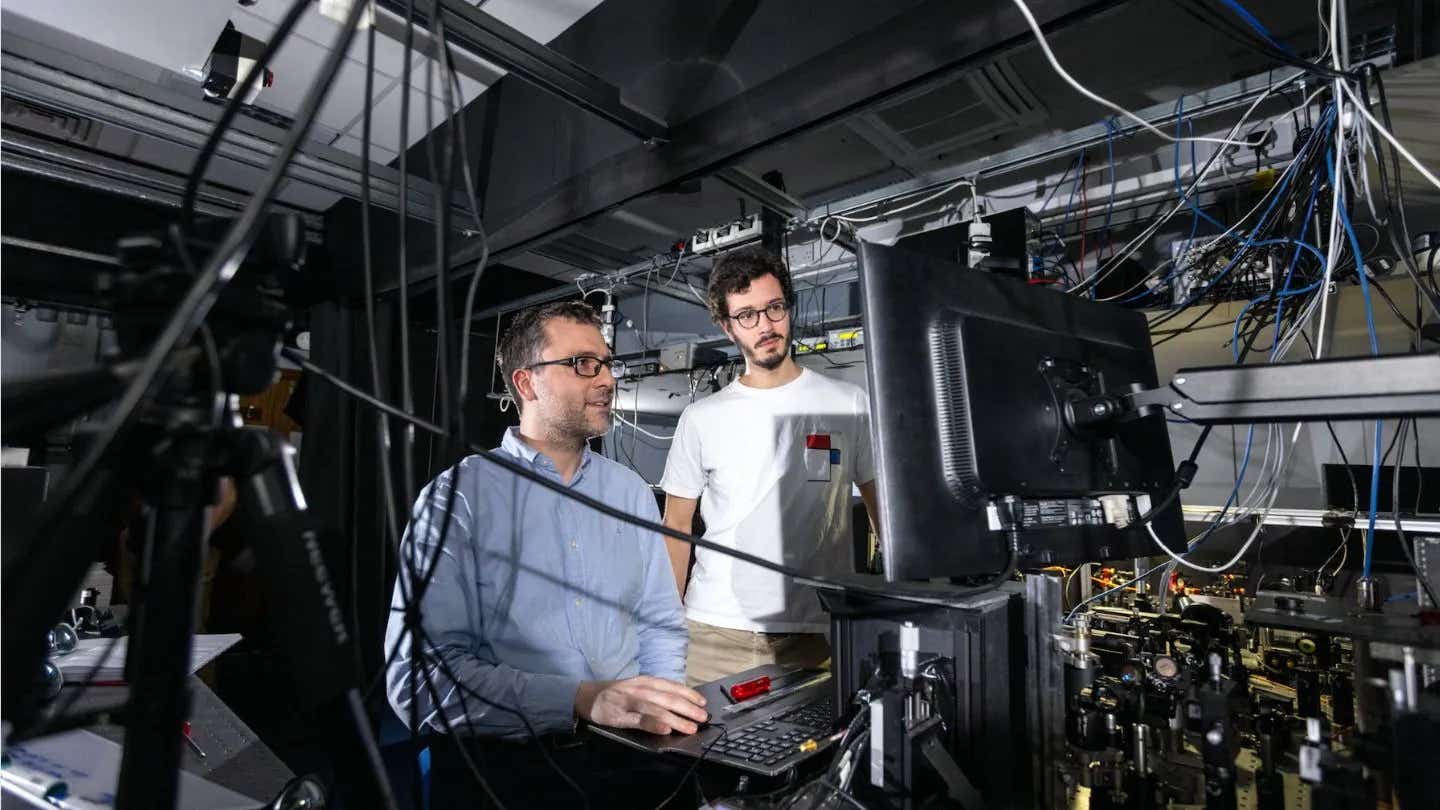
Professor Riccardo Sapienza and Sir John Pendry of the Department of Physics at Imperial College London. (CREDIT: ICL)
A team of physicists has reimagined one of science’s most iconic experiments—but in the dimension of time. Researchers at Imperial College London have taken the classic double-slit test and turned it into a time-based experiment, opening new frontiers in quantum physics.
Professor Riccardo Sapienza led the effort from the Department of Physics. His team explored how light interacts with a material that can change its optical properties in just a few femtoseconds. That’s one quadrillionth of a second—fast enough to catch light in the act of changing.
The original double-slit experiment, first performed by Thomas Young in 1801, showed that light behaves as a wave. Later, other versions revealed its dual nature, proving light can act like both waves and particles. This duality became a key piece in the puzzle of quantum mechanics.
In Young’s experiment, a beam of light passed through two physical slits and formed an interference pattern on a screen. The alternating light and dark bands confirmed that light can interfere with itself—something only waves do. This changed the way scientists think about particles like electrons and atoms.
This latest twist on the experiment does away with slits altogether. Instead, the team used lasers to change the frequency of light itself. They targeted a thin film of indium-tin-oxide, a transparent material also used in smartphone screens, and altered its optical traits with pinpoint timing.
The film’s reflectivity changed so quickly that it modified the color of the light, creating new patterns. These patterns weren’t spatial—they happened in time. The light’s frequencies clashed and overlapped, creating effects similar to those seen in classic interference.
Their findings, published in Nature Physics, have both deep scientific and real-world value. “This experiment reveals more about the fundamental nature of light while serving as a stepping-stone to creating materials that can control light in both space and time,” Sapienza said. Such control could spark major advances in everything from telecom to medical imaging.
One exciting outcome of the study is the possibility of new forms of spectroscopy. These methods could measure the structure of light pulses in time, down to the duration of a single wave cycle. That kind of resolution was unthinkable until now.
Related Stories
“The double time slits experiment opens the door to a whole new spectroscopy capable of resolving the temporal structure of a light pulse on the scale of one period of the radiation,” said co-author Professor Sir John Pendry. This leap in precision may help unlock details hidden in the shortest flashes of light.
The implications extend far beyond theoretical physics. The ability to precisely control the timing and frequency of light could transform telecommunications by enabling faster, more reliable data transmission. With improved optical switches, future communication networks could achieve higher speeds and efficiency, ultimately leading to advancements in internet connectivity and other technologies.
This research not only expands our understanding of quantum physics but also paves the way for transformative applications that could shape the future of technology and science.
In computing, the development of metamaterials that control light could result in optical processors that are faster and more energy-efficient than traditional electronic processors.
Optical computing, where light replaces electricity, has long been a goal for researchers because it promises higher data processing speeds with less energy consumption. Such technology could revolutionize computing by making devices more powerful while reducing their environmental impact.
Medical technology is another field that stands to benefit from these discoveries. Light’s potential to be precisely controlled in both space and time could lead to more advanced imaging tools for diagnostics and treatment.
This might allow for earlier detection of diseases or the ability to precisely target and destroy cancer cells without harming surrounding tissue, reducing side effects in treatments like chemotherapy.
The experiment also lays the foundation for further research into "time crystals," which are materials that have repeating structures not just in space but also in time.
According to co-author Professor Stefan Maier, "The concept of time crystals has the potential to lead to ultrafast, parallelized optical switches." These time crystals might help researchers achieve even more refined control over light, opening new avenues for technological advancement.
The significance of this experiment extends beyond telecommunications, computing, and medicine. Metamaterials like the ones used in this research could have broader applications in industries such as energy, transportation, aerospace, and defense.
For instance, controlling light at such a fine level might enable more efficient energy systems or advanced sensor technologies for aircraft and vehicles. Even black hole physics could be explored through these new quantum experiments, adding to the wide-ranging impact of this research.
As technology advances, the role of metamaterials and quantum physics will become increasingly critical. The ability to manipulate light in space and time holds the promise of reshaping how we interact with the world, offering faster, more efficient, and more precise tools across industries.
This breakthrough by the Imperial College London team marks a significant leap in that direction, showing how curiosity-driven research can lead to innovations that ripple through many fields.
As Professor Sapienza stated, this experiment is just the beginning: "Our work serves as a stepping-stone to creating the ultimate materials." With further exploration, these innovations could lead to entirely new ways of understanding and controlling light, providing tools that change everything from the devices in our pockets to the way we diagnose and treat diseases.
Note: Materials provided above by The Brighter Side of News. Content may be edited for style and length.
Like these kind of feel good stories? Get The Brighter Side of News' newsletter.
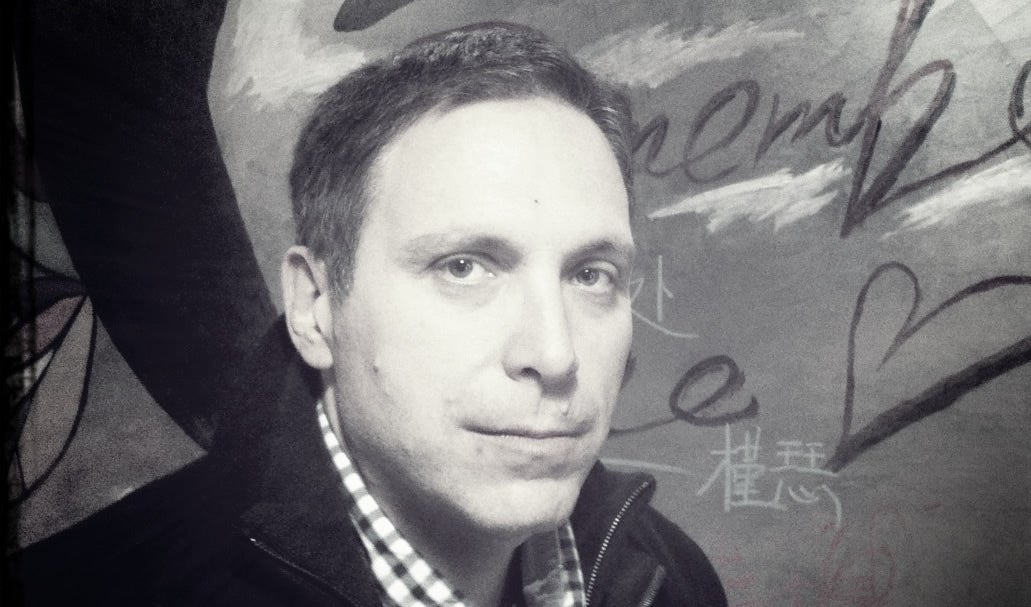
Joseph Shavit
Head Science News Writer | Communicating Innovation & Discovery
Based in Los Angeles, Joseph Shavit is an accomplished science journalist, head science news writer and co-founder at The Brighter Side of News, where he translates cutting-edge discoveries into compelling stories for a broad audience. With a strong background spanning science, business, product management, media leadership, and entrepreneurship, Joseph brings a unique perspective to science communication. His expertise allows him to uncover the intersection of technological advancements and market potential, shedding light on how groundbreaking research evolves into transformative products and industries.