Scientists create world’s first black hole bomb in the lab
When British physicist Roger Penrose suggested in 1969 that energy could be pulled from a rotating black hole, it sparked a half-century of exploration.
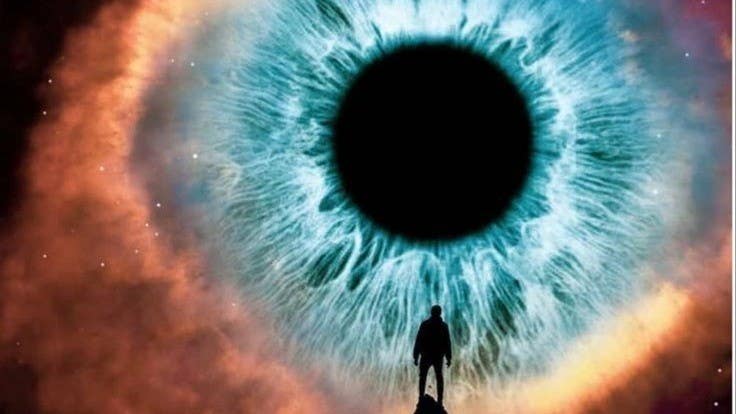
Physicists have successfully built a “black hole bomb” analog in the lab. (CREDIT: CC BY-SA 4.0)
When British physicist Roger Penrose suggested in 1969 that energy could be pulled from a rotating black hole, it sparked a half-century of exploration. Penrose proposed that inside the ergosphere—the area just outside a black hole's event horizon—particles could split. One fragment could fall into the black hole carrying negative energy, while the other could escape, boosted with extra energy.
Though strange, the idea fits perfectly with general relativity. The absorbed fragment’s negative energy actually reduces the black hole’s mass and rotation, allowing the escaping fragment to gain energy without breaking the laws of physics.
A few years later, Belarusian physicist Yakov Zel’dovich wondered if you could mimic this cosmic trick without needing a real black hole. In 1971, he theorized that a rotating metal cylinder could amplify waves in a similar way. If a wave with angular momentum hit a fast-spinning cylinder, it could get reflected back stronger—stealing a little energy from the cylinder’s rotation.
Zel’dovich’s prediction was bold. He even suggested that if the cylinder was surrounded by a mirror, it could trigger a runaway feedback loop, amplifying small noise into a powerful signal. This mirror setup, creating a “black hole bomb,” was later formalized by Press and Teukolsky. Yet for decades, no one had been able to confirm it with electromagnetic waves. Until now.
First Laboratory "Black Hole Bomb"
Researchers at the University of Southampton, led by Hendrik Ulbricht and including Marion Cromb, have finally achieved what Zel’dovich envisioned. In a new experiment, scientists built a rotating system using a simple aluminum cylinder and a three-phase magnetic field. The setup created the special conditions where the cylinder’s rotation could amplify electromagnetic waves.
They didn’t stop there. By adding a resonant circuit—a kind of electromagnetic "mirror"—around the cylinder, the team watched something extraordinary. From only random background noise, electromagnetic waves began to grow stronger and stronger. This runaway growth perfectly mimicked what would happen near a real black hole bomb.
“We’re basically generating a signal from noise, and that is the same thing that happens in the black hole bomb proposal,” said Ulbricht.
The experiment showed for the first time that spontaneous amplification and exponential growth from noise, predicted for rotating black holes, really can happen—even in a laboratory toy model.
Related Stories
Unraveling the Zel’dovich Effect
The Zel’dovich effect works through a fascinating twist of physics. If a rotating object moves faster than the frequency of incoming radiation, the waves’ energy changes in a surprising way. They undergo the rotational Doppler effect, a cousin of the familiar linear Doppler effect that makes an ambulance siren rise or fall in pitch as it moves past you.
As Cromb explained, "The twisted sound waves change their pitch when measured from the point of view of the rotating surface. If the surface rotates fast enough then the sound frequency can go from a positive frequency to a negative one, and in doing so steal some energy from the rotation of the surface."
In previous studies, the team had tested this effect using sound waves. They bounced sound off a spinning disc and saw the energy shift predicted by theory. But applying the same principle to electromagnetic waves required overcoming tougher challenges.
By spinning the aluminum cylinder quickly enough, they caused the incoming twisted electromagnetic fields to become rotationally Doppler-shifted into negative frequency territory. Negative frequency means negative absorption, which translates into amplification.
Vitor Cardoso, a physicist at the University of Lisbon, marveled at the results. "You throw a low-frequency electromagnetic wave against a spinning cylinder, who would think that you get back more than what you threw in? It’s totally mind boggling," he told New Scientist.
Connecting Black Holes, Dark Matter, and Particle Detection
The experiment’s implications stretch far beyond the lab. Physicists believe superradiance—the process at the heart of the black hole bomb—could help detect unknown particles, including candidates for dark matter.
Cardoso explained, "Superradiance is turning black holes into particle detectors, and much better particle detectors than [the Large Hadron Collider at] CERN can be for this type of dark matter."
If lightweight, unknown particles exist, they could cluster around spinning black holes, siphoning energy through superradiance. As a result, scientists might detect strange gravitational waves or even notice black holes spinning down faster than expected.
The laboratory confirmation of superradiant amplification gives researchers more confidence to search for these signs in real black hole systems across the universe.
Echoes of a Black Hole Bomb
In their study posted to the pre-print server arXiv, the Southampton team described how the aluminum cylinder did more than just amplify incoming electromagnetic waves. It became unstable and acted as a self-sustaining generator. Once noise seeded the runaway amplification, the energy grew exponentially.
The experiment even captured another important detail. As the cylinder’s rotational energy decreased, the amplification eventually stopped, just like Cardoso and colleagues predicted would happen near real black holes when they lose too much spin.
The new experiment follows the chain of ideas stretching back to Penrose, Zel’dovich, and Press and Teukolsky. By translating the mysteries of black hole physics into a tabletop laboratory model, it opens new paths for understanding deep cosmic processes.
In the future, researchers hope to go even further. One major goal is to observe spontaneous electromagnetic wave generation seeded by quantum vacuum fluctuations. Achieving that will require refining the experimental setup, but it no longer seems like a distant dream.
As the Southampton team writes, "Based on the results presented here, this now remains a purely technological (even if very hard) feat."
Thanks to the simple tools of rotating cylinders, mirrors, and clever electromagnetic fields, scientists are beginning to tame the exotic physics of the universe’s most extreme objects—all without leaving Earth.
Note: The article above provided above by The Brighter Side of News.
Like these kind of feel good stories? Get The Brighter Side of News' newsletter.
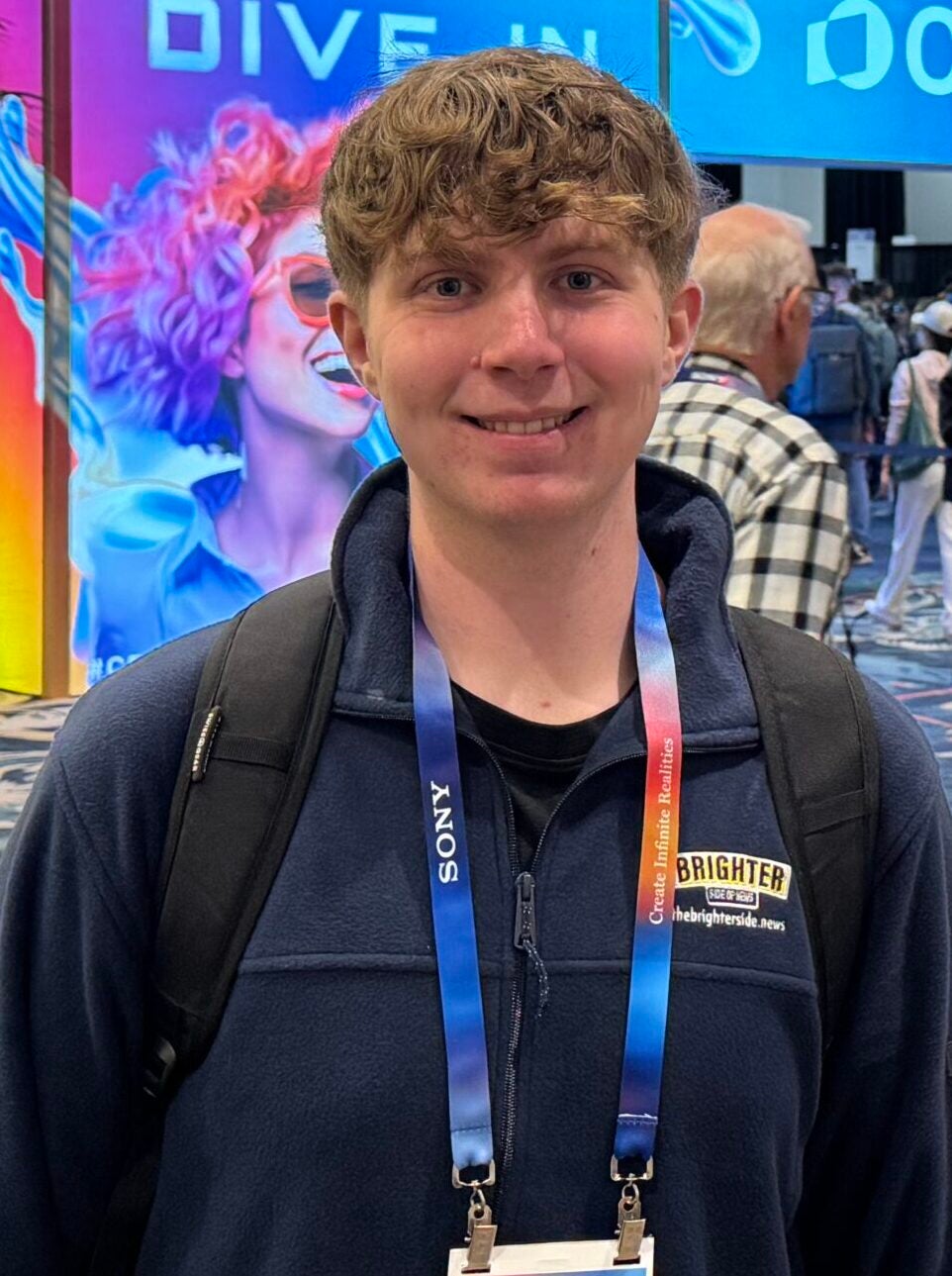
Joshua Shavit
Science & Technology Writer | AI and Robotics Reporter
Joshua Shavit is a Los Angeles-based science and technology writer with a passion for exploring the breakthroughs shaping the future. As a contributor to The Brighter Side of News, he focuses on positive and transformative advancements in AI, technology, physics, engineering, robotics and space science. Joshua is currently working towards a Bachelor of Science in Business Administration at the University of California, Berkeley. He combines his academic background with a talent for storytelling, making complex scientific discoveries engaging and accessible. His work highlights the innovators behind the ideas, bringing readers closer to the people driving progress.