New discovery upends current models of star and planet formation
When a star is born, it doesn’t emerge in isolation. Instead, it forms inside a large, chaotic cloud of gas and dust.
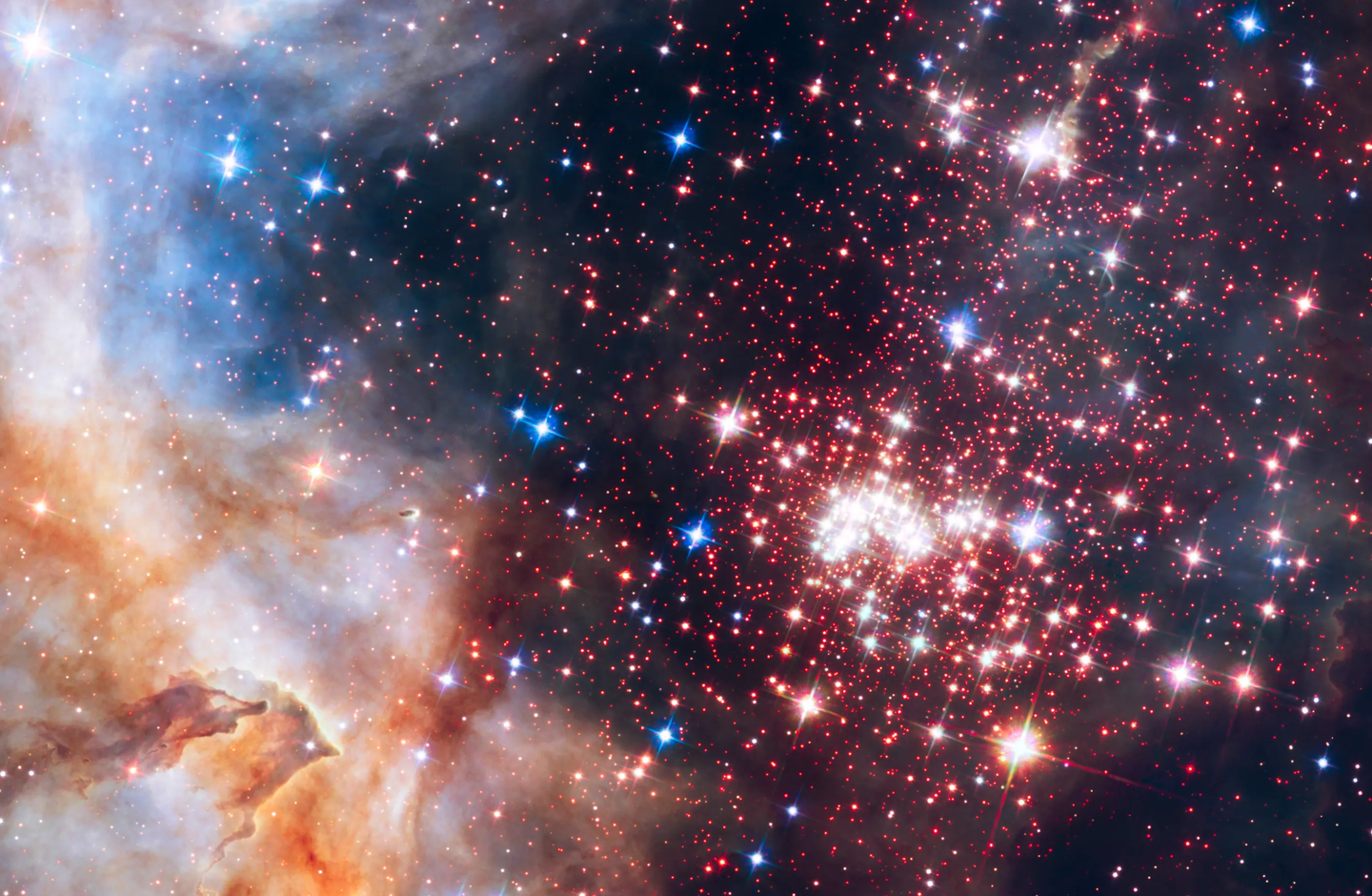
A new theory reshapes how stars grow their planet-making disks. (CREDIT: NASA, ESA, the Hubble Heritage Team (STScI/AURA), A. Nota (ESA/STScI), and the Westerlund 2 Science Team)
When a star is born, it doesn’t emerge in isolation. Instead, it forms inside a large, chaotic cloud of gas and dust. Around it, a flat disk of spinning material takes shape. This protoplanetary disk is where planets are made. Until recently, scientists believed this disk was a leftover remnant from the birth of the star—what was left behind when the collapsing cloud had finished feeding the central star.
That traditional view sees the disk as a sealed system with limited mass and spin. The problem with this idea is that it doesn't explain what astronomers have been seeing. Observations, especially those from powerful telescopes like ALMA, show young stars with disks that are far too large, too massive, or too long-lived to match old models. The math just didn’t add up.
A groundbreaking study published in Nature Astronomy and led by Paolo Padoan, a research professor at the Institute of Cosmos Sciences of the University of Barcelona and visiting scientist at Dartmouth College, offers a new way to look at how these disks grow and evolve. His work shows that the surrounding environment plays a much bigger role than anyone thought.
A New Way to Build Planetary Disks
Rather than assuming that the disk finishes forming once the star itself has come together, the study proposes that young stars keep gathering gas and dust from their birth cloud long after they ignite. This ongoing collection of material happens through a process called Bondi-Hoyle accretion. It allows the star to keep pulling in matter from its surroundings, adding more material to its disk.
That means these disks aren’t just passive leftovers—they’re active systems that grow over time, fed by the star’s gravity. This fresh view changes how we think about both how planets form and how long the disks last.
“Stars are born in groups or clusters within large gas clouds and can remain in this environment for several million years after their birth,” says Padoan. “After a star forms, its gravity can capture more material from the parental gas cloud, which is not enough to change the star’s mass significantly but enough to restructure its disk.”
Related Stories
In other words, the environment isn't done shaping the star system even after the star itself has lit up. The cloud around it continues to feed the disk with new material.
Spinning Bigger Disks with Turbulent Gas
Disks need more than just mass to grow—they need spin. This spin, or angular momentum, is what keeps the disk wide and flat, and it’s also what drives planet formation. Before this study, scientists didn’t believe Bondi-Hoyle accretion could supply enough angular momentum to match what telescopes observed.
But that assumption ignored a key part of the picture: turbulence.
Gas clouds in space aren’t still. They swirl and tumble with supersonic winds and violent shifts in density. That chaos, it turns out, matters a lot. The study reveals that density fluctuations in turbulent gas can increase the angular momentum delivered to a star’s disk by a huge amount—enough to match or even exceed what’s been seen in young stellar systems.
Padoan’s team used a mix of math and advanced computer simulations to prove their point. They created models showing how different sources of angular momentum arise. One source is the rotation of gas itself. But the larger and more important source, especially in supersonic turbulence, comes from how the densest parts of the cloud shift and pull on the disk.
These density fluctuations offset the center of mass, changing how material flows onto the disk. In regular, smooth gas (what physicists call incompressible), this offset wouldn’t matter. But in turbulent gas, it makes a major difference.
This helps explain why older models underestimated how much spin a disk could gain. The models looked only at smooth flows or local rotation but skipped over the more powerful contribution from chaotic density shifts.
Solving Long-Standing Mysteries
The updated model clears up several problems that have puzzled astronomers for years. For example, many disks were thought to be too large or last too long to fit the standard theory. Others showed strange tilts or misalignments, with orbits of planets pointing in unexpected directions. Some star systems had disks that didn’t match the expected ratio of size to stellar mass.
All of these issues can now be explained by the new model. Because the disk is still pulling in new material from its surroundings, it can grow larger, live longer, and end up with more complicated structures than earlier theories allowed. The incoming gas also brings new spin, which can change the direction of the disk and explain misalignments in both disks and exoplanet orbits.
In short, the traditional view of isolated disks just doesn’t fit the evidence. Bondi-Hoyle accretion offers a better match to what’s being observed in space.
“Comparing the observable data from the simulations with real observations is crucial to validate the simulations,” says Veli-Matti Pelkonen, a member of Padoan’s team at ICCUB. “However, simulations allow us to go beyond the observables to the underlying density, velocity and magnetic field structures, and to follow them in time.”
Pelkonen believes that as supercomputers grow more powerful, the ability to simulate more complex systems will only improve. This, in turn, will help connect theoretical models more closely to what astronomers actually see through telescopes.
A Better Path to Finding Habitable Worlds
Understanding how protoplanetary disks grow doesn’t just matter for learning about stars. It also changes how scientists think about where and how planets form—including those that might be like Earth.
If a disk is constantly gaining new material, it can stay active longer. This gives planets more time to form and more raw materials to use. The extended disk lifetime may improve the chances of forming rocky planets in stable orbits with the right conditions for life.
Since disks are shaped by their environment, this also suggests that the search for habitable worlds should look closely at the birthplaces of stars. The kind of gas cloud a star forms in might affect the kind of planetary system it can support.
It’s a reminder that nothing in space happens in isolation. From the first spark of star formation to the slow shaping of a solar system, everything is tied together by the environment around it.
As telescopes like ALMA and the James Webb Space Telescope continue to look deeper into the early lives of stars, more discoveries like these are expected. By combining high-resolution observations with powerful simulations, astronomers are rewriting the story of how planets—and maybe life—begin.
Note: The article above provided above by The Brighter Side of News.
Like these kind of feel good stories? Get The Brighter Side of News' newsletter.
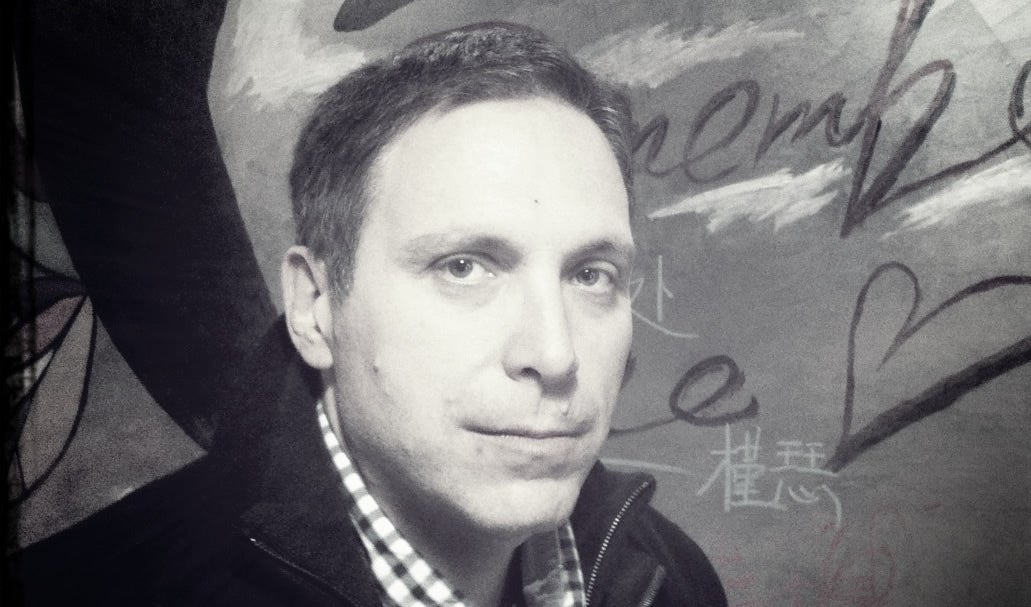
Joseph Shavit
Head Science News Writer | Communicating Innovation & Discovery
Based in Los Angeles, Joseph Shavit is an accomplished science journalist, head science news writer and co-founder at The Brighter Side of News, where he translates cutting-edge discoveries into compelling stories for a broad audience. With a strong background spanning science, business, product management, media leadership, and entrepreneurship, Joseph brings a unique perspective to science communication. His expertise allows him to uncover the intersection of technological advancements and market potential, shedding light on how groundbreaking research evolves into transformative products and industries.