New quantum gravity discovery could unite quantum mechanics and relativity
Researchers observing rare cosmic events have uncovered mechanisms that could reshape both industry and our view of the universe.
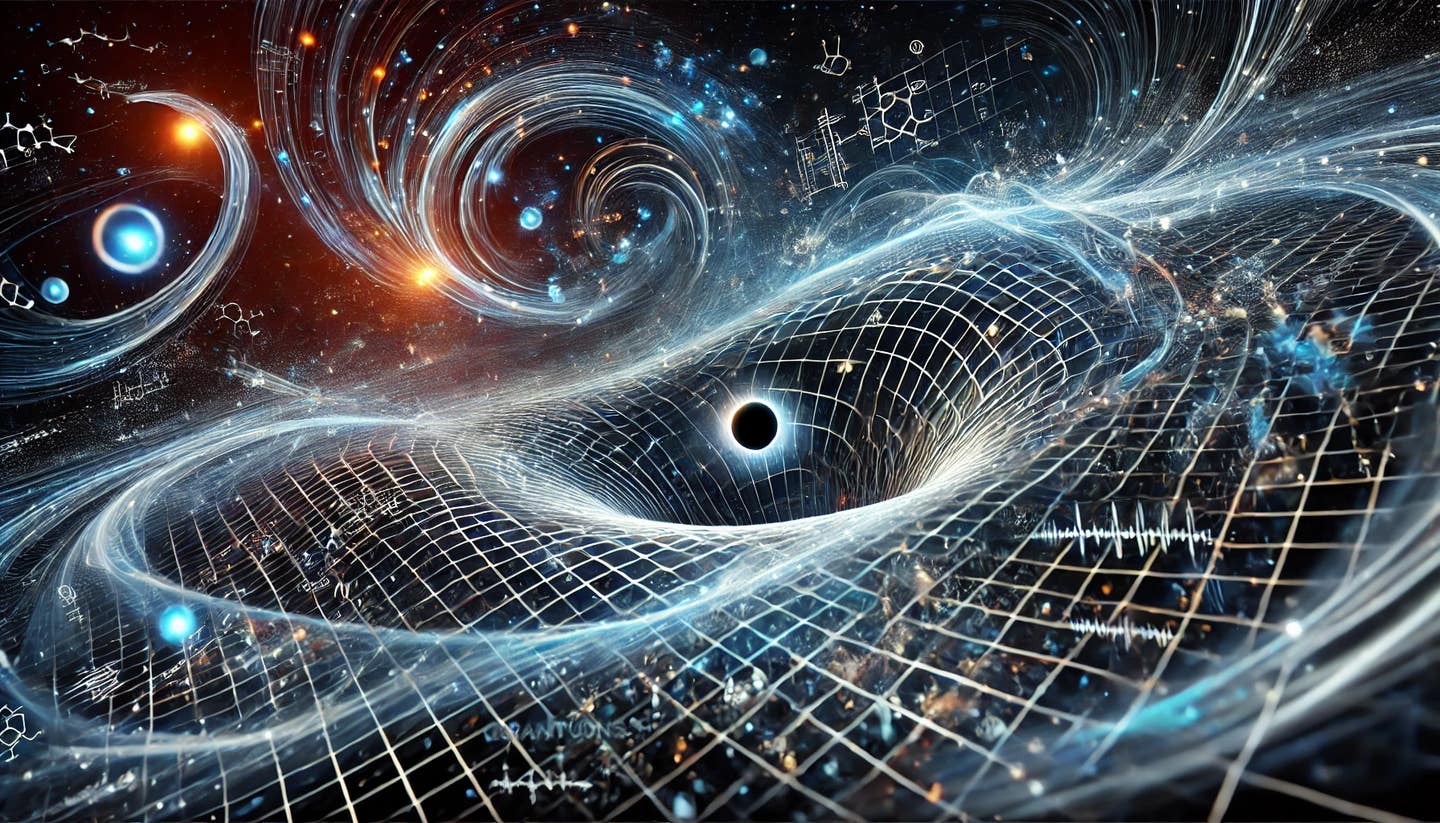
Explore groundbreaking advances reshaping science—from the intricate molecular dance within MOFs revolutionizing chemical separation to the startling visibility of singularities unlocking the secrets of the cosmos. (CREDIT: CC BY-SA 4.0)
Breakthroughs in science often begin with a simple question and years of tireless exploration. From the way tiny molecules slip through microscopic pores to the staggering behavior of matter near cosmic singularities, each new insight opens doors to discoveries with real-world impact.
Two recent advances—one in nanoscale chemistry and another in astrophysics—are making waves. Scientists studying the movement of molecules in porous materials and researchers observing rare cosmic events have uncovered mechanisms that could reshape both industry and our view of the universe.
The Promise of Molecular Diffusion
One of the most promising fields in material science centers on molecular diffusion. This is the way molecules move through small, confining spaces—a key process behind technologies like gas separation, catalysis, and energy storage. Materials called MOFs, short for metal-organic frameworks, have emerged as powerful tools because of their flexible structure and tunable chemistry.
Yet predicting how molecules behave inside these frameworks isn’t simple. Pore size, shape, chemical reactivity, and even how the material flexes all play a role. Studying these factors one by one has been manageable. But understanding how they work together to control molecular flow remains a major hurdle for material designers.
To address this, researchers turned to a group of chemicals used in making lubricants, pesticides, and plastics. By looking at how two similar molecules—1-bromopropane (1BP) and 2-bromopropane (2BP)—travel through a MOF, they aimed to decode how to control and even reverse diffusion behavior. These insights could lead to better industrial separation processes.
The Crafting of Metal-Organic Frameworks
The results, published in Nature Communications, revealed a surprising outcome. The scientists used a carefully crafted MOF thin film and demonstrated that it’s possible to flip the natural preference of one isomer over the other. This reversal in diffusion selectivity was achieved by tuning the way molecules interact inside the structure.
Their MOF was built with copper-based paddle-wheel nodes linked by two organic molecules—bdc and azbpy. This created a pillared-layer structure with two unique pore windows: one just 7.3 by 4.3 angstroms and another measuring 9.7 by 6.9 angstroms. These dimensions were fine-tuned to separate the isomers with high precision.
To ensure the MOF's channels lined up exactly, the team used a method called layer-by-layer epitaxial growth. The final structure was then verified using tools like X-ray diffraction and electron microscopy. These imaging techniques confirmed the material’s design and the alignment of its pores, key to its selective performance.
Related Stories
Combining molecular simulations with kinetic experiments revealed that chemical interactions between the adsorbate (the isomers) and the MOF framework could modulate diffusion rates.
Researchers found that the orientation of nanochannels and the chemical functionality on pore surfaces worked synergistically to control molecular movement. Dynamic interactions allowed the team to reverse the diffusion selectivity of 1BP and 2BP, demonstrating how molecular pathways could be manipulated at an atomic scale.
Precision Chemical Separations
These findings hold significant implications for industrial processes requiring precise chemical separations. "Dynamic chemical interactions within MOFs not only regulate molecular diffusion but also offer a pathway to fine-tune material properties for specific applications," the researchers noted. By leveraging these interactions, MOFs could make separation technologies more energy-efficient and environmentally sustainable.
On a vastly different scale, astrophysicists are challenging conventional understandings of the universe by investigating singularities—points of infinite density resulting from gravitational collapse.
Traditionally, singularities are thought to exist only within black holes, concealed behind event horizons that prevent observation. However, recent research suggests the existence of naked or visible singularities, which could offer unprecedented insights into the universe's fundamental structure.
In related research, Professors Pankaj Joshi and Sudip Bhattacharyya proposed that gravitational collapse in the early universe could have produced primordial naked singularities (PNaSs). Unlike black holes, PNaSs are not shrouded by event horizons, making them observable. These singularities could represent a significant fraction of dark matter, which constitutes about 25% of the universe’s total mass-energy content.
Black Holes and Naked Singularities
Their research builds on earlier hypotheses by Stephen Hawking and others, who suggested that quantum fluctuations in the early universe could lead to the formation of primordial black holes. Joshi and Bhattacharyya extended this idea, showing that sufficiently dense regions of matter could form PNaSs instead.
These naked singularities may provide a rare opportunity to study quantum gravity—a theoretical framework that seeks to reconcile quantum mechanics with general relativity. "Understanding quantum gravity is one of the last major frontiers of physics," the researchers stated. "PNaSs offer a unique opportunity to observe phenomena that are otherwise inaccessible in black holes."
If PNaSs form a substantial portion of dark matter, they could fundamentally change how we perceive the universe. Unlike traditional dark matter, which interacts only through gravity, PNaSs could be directly observed and studied. This accessibility opens new avenues for investigating the quantum effects of gravity, which could help physicists develop a unified theory of the universe.
Common Pursuit of Complex Systems
Despite their differences in scale and subject matter, the studies of molecular diffusion in MOFs and cosmic singularities share a common pursuit: understanding complex systems to uncover their underlying principles. Advances in computational modeling and experimental techniques have been critical to both endeavors.
In the realm of MOFs, molecular dynamics simulations provide valuable insights into diffusion pathways and chemical interactions. These tools allow researchers to predict how structural changes in the MOF framework affect molecular movement.
Similarly, in astrophysics, theoretical models and simulations help visualize phenomena like gravitational collapse and quantum fluctuations, guiding researchers in exploring the extreme conditions of the universe.
Both fields also highlight the importance of interdisciplinary collaboration. Chemists, physicists, and computational scientists work together to enhance the functionality of MOFs, while astrophysicists draw on quantum theory and general relativity to develop models of singularities. Such collaborations accelerate progress and open new possibilities for scientific discovery.
Potential Real-World Applications
The potential applications of these findings are vast. MOFs with tunable diffusion properties could revolutionize industries reliant on chemical separations, such as petrochemicals, pharmaceuticals, and environmental remediation. Their energy efficiency and adaptability make them ideal for developing sustainable technologies, such as carbon capture and hydrogen storage.
In astrophysics, PNaSs could hold the key to understanding dark matter and quantum gravity, two of the most profound mysteries in modern science. These singularities may reveal quantum effects in gravitational systems, offering insights that were previously considered unattainable. They could also serve as natural laboratories for testing proposed theories of quantum gravity, bridging the gap between quantum mechanics and general relativity.
Both studies underscore the value of pushing scientific boundaries. By delving into the nanoscale world of MOFs and the cosmic realm of singularities, researchers are unlocking the secrets of the universe at both ends of the scale. These discoveries not only advance scientific knowledge but also lay the groundwork for transformative technologies and theoretical breakthroughs.
As science continues to explore the unknown, the lines between disciplines blur, and the potential for discovery grows. Whether in the confined pores of a MOF or the infinite density of a singularity, the quest for understanding unites us in our search for meaning in the natural world.
Note: Materials provided above by The Brighter Side of News. Content may be edited for style and length.
Like these kind of feel good stories? Get The Brighter Side of News' newsletter.
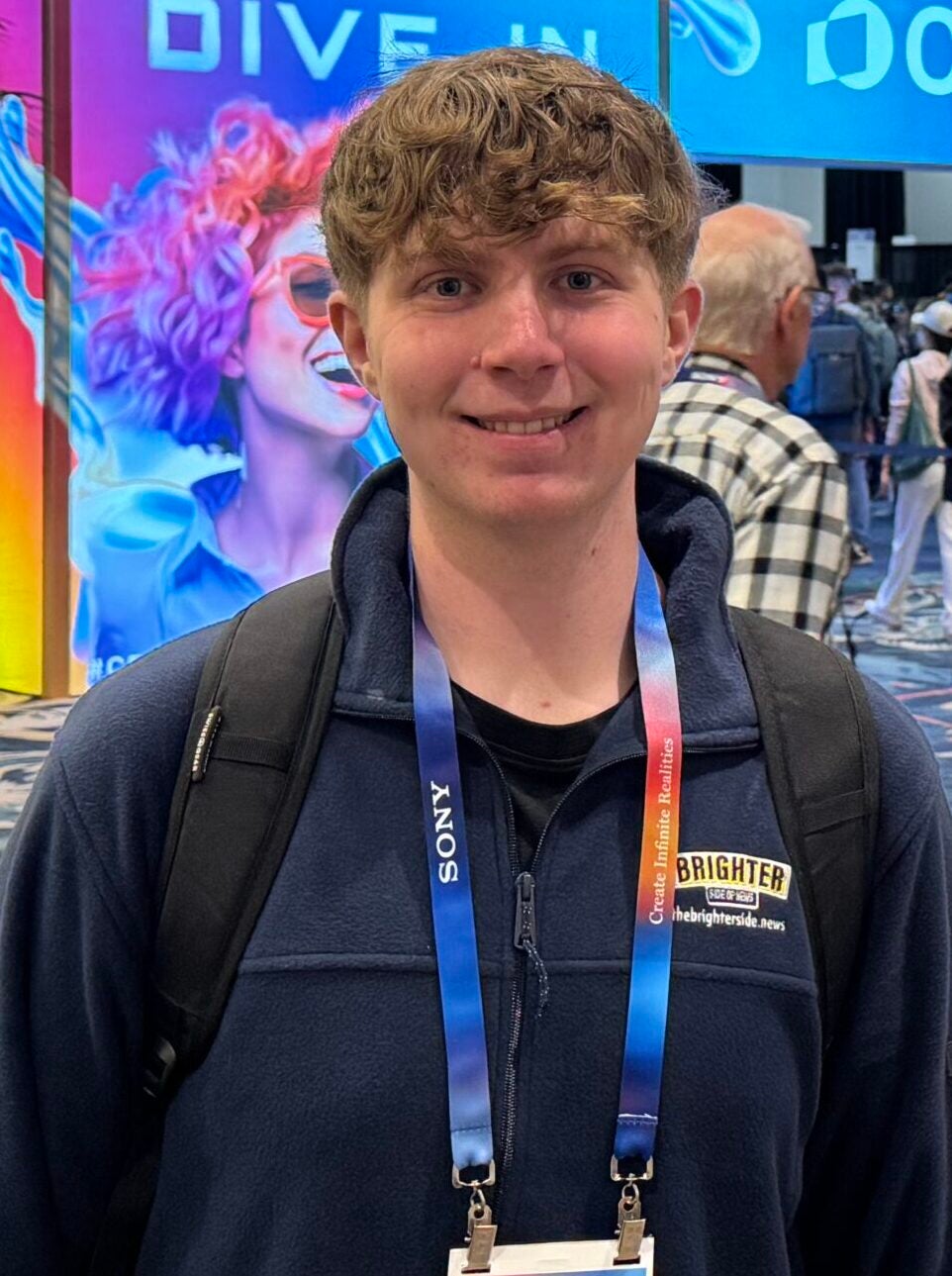
Joshua Shavit
Science & Technology Writer | AI and Robotics Reporter
Joshua Shavit is a Los Angeles-based science and technology writer with a passion for exploring the breakthroughs shaping the future. As a contributor to The Brighter Side of News, he focuses on positive and transformative advancements in AI, technology, physics, engineering, robotics and space science. Joshua is currently working towards a Bachelor of Science in Business Administration at the University of California, Berkeley. He combines his academic background with a talent for storytelling, making complex scientific discoveries engaging and accessible. His work highlights the innovators behind the ideas, bringing readers closer to the people driving progress.