Scientists link dark energy to alien life in the multiverse
The vastness of the cosmos hides more than distant stars—it conceals the very forces that shape reality.
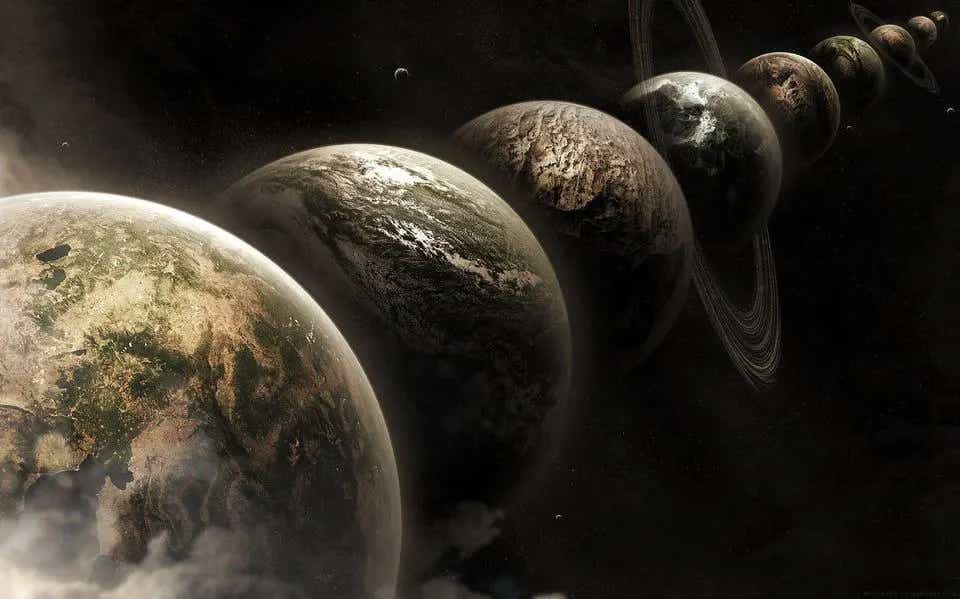
Dark energy density and star formation rates shape the Universe’s potential for intelligent life in our cosmos and beyond. (CREDIT: Lee Davy of flickr)
The vastness of the cosmos hides more than distant stars—it conceals the very forces that shape reality. Astronomers have long searched for answers beyond what telescopes can see, driven by questions about how galaxies form and evolve in the fabric of space.
Even as science advances, some cosmic puzzles remain. Processes like how stars are born, how gas moves through space, and how galaxies respond to internal pressures are still not fully clear. Adding to this mystery is dark energy—a strange force pushing the Universe to expand faster over time. This phenomenon may hold key clues about the potential for life to exist.
Researchers at Durham University have stepped into this unknown with bold new ideas. Their latest study unveils a model that tries to estimate how likely intelligent life is—not just in our own Universe, but across possible multiverses.
The approach takes cues from the famous Drake Equation, which once guided the search for alien civilizations. This updated model focuses on how the density of dark energy and the pace of star formation work together to influence the chance of life emerging.
A Cosmological Framework for Star Formation
The theory is rooted in the Cold Dark Matter (CDM) paradigm, a leading model for explaining the Universe’s large-scale structure. It proposes that galaxies form from dark matter halos collapsing under gravity, starting from nearly even conditions shortly after the Big Bang.
N-body simulations and analytical tools have helped refine this picture. They map out how these dark matter halos come together. Still, the behavior of ordinary matter—gas, stars, and the energetic forces they produce—remains a harder piece to solve.
Early attempts at modeling focused on simple ideas: how long gas takes to cool and how fast it turns into stars. But they often skipped over the powerful feedback effects from stars and supermassive black holes at galactic centers.
Related Stories
Later models improved on this, adding layers of complexity. They examined how stars and black holes grow together, how they influence one another, and how they shape the galaxies that contain them.
Hydrodynamical simulations took things even further. These advanced tools recreate the dance between regular matter and dark matter, accounting for the explosive effects of supernovae and the intense energy from black hole jets.
Still, these simulations rely on “subgrid” models—rough methods used to approximate small-scale processes that can’t be directly resolved. Because of this, the accuracy of their predictions across different cosmic setups remains uncertain.
Dark Energy and Its Paradoxes
Dark energy comprises over two-thirds of the Universe's total energy and governs its accelerated expansion. Despite its pivotal role, dark energy's physical nature remains enigmatic.
A prevailing hypothesis links it to the quantum vacuum's energy, yet theoretical calculations yield discrepancies spanning orders of magnitude. Efforts to reconcile this "cosmological constant problem" include invoking scalar fields, modified gravity theories, or multiverse scenarios.
One puzzle, the "why-now problem," questions why dark energy began dominating the Universe's expansion only recently, coinciding with the Sun's formation.
Some theories suggest anthropic reasoning, where cosmic parameters are constrained by the conditions necessary for observers to exist. In this context, multiverse models propose an ensemble of universes, each with different physical constants.
A New Model for Intelligent Life
Durham University's research builds on these ideas, introducing a model that links dark energy density and star formation rates to the emergence of life. Unlike the Drake Equation, which estimates intelligent civilizations in the Milky Way, this approach calculates the relative probability of life across hypothetical universes.
The team explored the fraction of ordinary matter converted into stars over cosmic history under varying dark energy densities. In our Universe, this fraction is approximately 23%. However, the model predicts that a universe with a higher dark energy density could achieve a star formation efficiency of 27%. This suggests our Universe, while life-supporting, may not maximize the conditions for life to develop.
"Understanding dark energy and its impact on our Universe is one of the biggest challenges in cosmology," said Dr. Daniele Sorini, lead researcher. "Surprisingly, we found that even significantly higher dark energy densities would still be compatible with life, suggesting we may not live in the most likely of universes."
Implications for Parallel Universes
The study's findings have profound implications for the concept of parallel universes. In stochastic inflation models, an infinite multiverse arises, with each "bubble universe" possessing unique physical constants. This ensemble provides a statistical framework for understanding why our Universe has its specific properties.
Anthropic reasoning, while controversial, gains traction as an explanatory tool in this context. Similar to the concept of habitable zones around stars, this approach considers conditions conducive to life. By exploring the astrophysics of star formation and large-scale structure evolution, researchers aim to identify universal parameters that enable life.
"It will be exciting to employ the model to explore the emergence of life across different universes," noted co-author Professor Lucas Lombriser of Université de Genève. "This could lead to a rethinking of fundamental questions about our own Universe."
Toward a Unified Understanding
The interplay between dark energy, star formation, and life's potential challenges scientists to expand theoretical and computational boundaries. Full hydrodynamical simulations offer detailed insights but are computationally intensive. Simplified analytical models, while less precise, provide intuitive frameworks for exploring cosmic evolution over vast timescales.
By combining these approaches, researchers hope to address longstanding questions about the Universe's fine-tuning. Why does our Universe support intelligent life? How do its parameters compare to hypothetical multiverses?
These investigations bridge astrophysics, cosmology, and the search for extraterrestrial life, offering a richer understanding of our place in the cosmos.
Note: Materials provided above by The Brighter Side of News. Content may be edited for style and length.
Like these kind of feel good stories? Get The Brighter Side of News' newsletter.
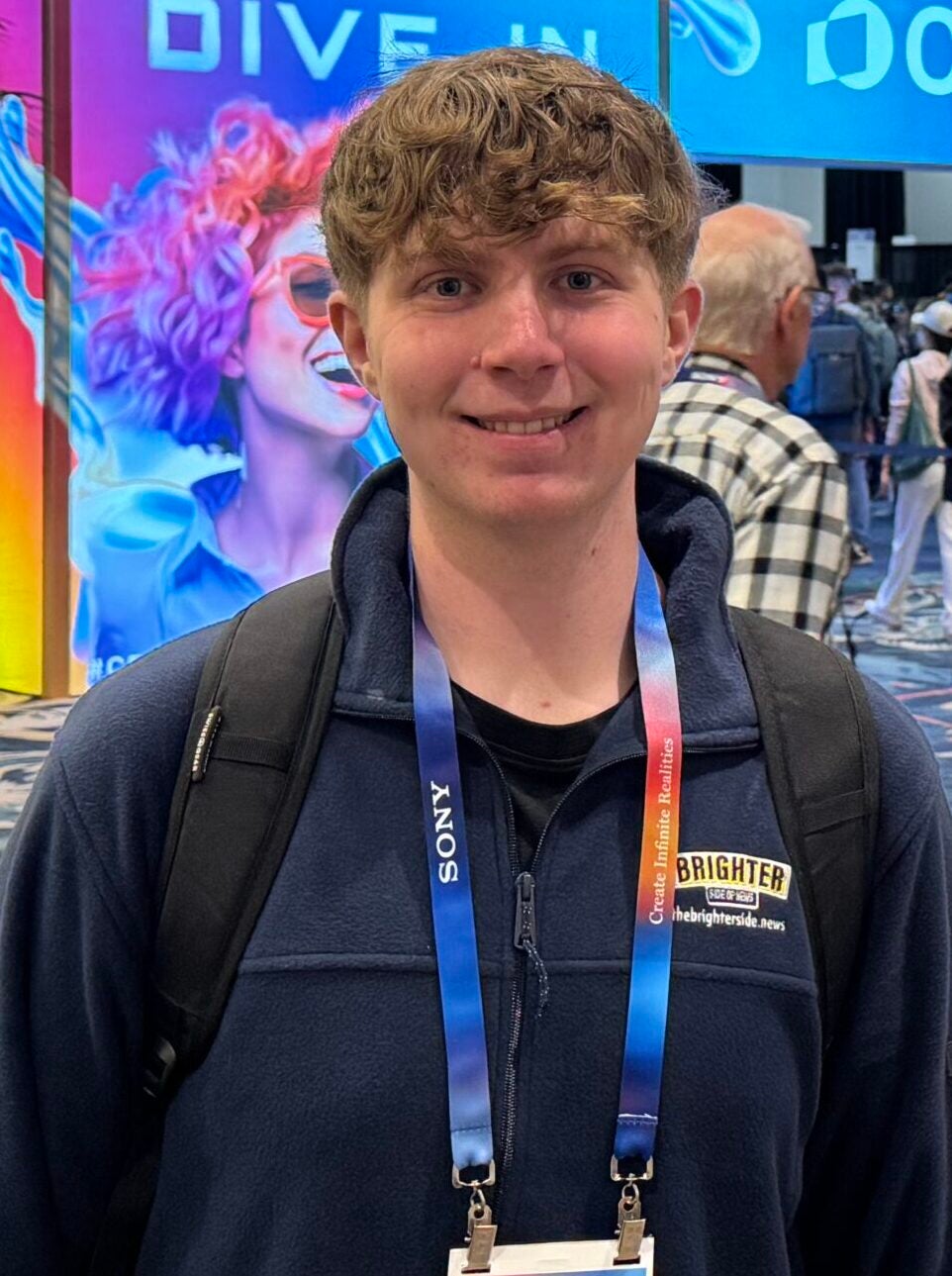
Joshua Shavit
Science & Technology Writer | AI and Robotics Reporter
Joshua Shavit is a Los Angeles-based science and technology writer with a passion for exploring the breakthroughs shaping the future. As a contributor to The Brighter Side of News, he focuses on positive and transformative advancements in AI, technology, physics, engineering, robotics and space science. Joshua is currently working towards a Bachelor of Science in Business Administration at the University of California, Berkeley. He combines his academic background with a talent for storytelling, making complex scientific discoveries engaging and accessible. His work highlights the innovators behind the ideas, bringing readers closer to the people driving progress.